- 1Centro de Fruticultura, Facultad de Ciencias Agropecuarias y Medioambiente, Universidad de La Frontera, Temuco, Chile
- 2Escuela de Agronomía, Pontificia Universidad Católica de Valparaíso, Quillota, Chile
- 3Departamento de Ciencias Agronómicas y Recursos Naturales, Facultad de Ciencias Agropecuarias y Medioambiente, Universidad de La Frontera, Temuco, Chile
- 4Departamento de Producción Agropecuaria, Facultad de Ciencias Agropecuarias y Medioambiente, Universidad de la Frontera, Temuco, Chile
- 5Department of Horticultural Sciences, University of Bonn, Bonn, Germany
- 6Facultad de Ciencias Agropecuarias y Medioambiente, Universidad de La Frontera, Temuco, Chile
Temperate fruit and nut crops require distinctive cold and warm seasons to meet their physiological requirements and progress through their phenological stages. Consequently, they have been traditionally cultivated in warm temperate climate regions characterized by dry-summer and wet-winter seasons. However, fruit and nut production in these areas faces new challenging conditions due to increasingly severe and erratic weather patterns caused by climate change. This review represents an effort towards identifying the current state of knowledge, key challenges, and gaps that emerge from studies of climate change effects on fruit and nut crops produced in warm temperate climates. Following the PRISMA methodology for systematic reviews, we analyzed 403 articles published between 2000 and 2023 that met the defined eligibility criteria. A 44-fold increase in the number of publications during the last two decades reflects a growing interest in research related to both a better understanding of the effects of climate anomalies on temperate fruit and nut production and the need to find strategies that allow this industry to adapt to current and future weather conditions while reducing its environmental impacts. In an extended analysis beyond the scope of the systematic review methodology, we classified the literature into six main areas of research, including responses to environmental conditions, water management, sustainable agriculture, breeding and genetics, prediction models, and production systems. Given the rapid expansion of climate change-related literature, our analysis provides valuable information for researchers, as it can help them identify aspects that are well understood, topics that remain unexplored, and urgent questions that need to be addressed in the future.
1 Introduction
Most temperate fruit and nut orchards are located at mid-latitudes between 30° and 50°, in both the Northern and Southern hemispheres (Rai et al., 2015). Most of these regions feature a warm temperate climate, which, according to the Köppen-Geiger classification system, is characterized primarily by Mediterranean-like climatic conditions with distinct dry-summer and wet-winter seasons (Kottek et al., 2006; Peel et al., 2007; Sarricolea et al., 2017). Globally, warm temperate climate regions represent 13.4% of the land area (Peel et al., 2007). The climate of these regions is well-suited for temperate fruit crops, which require, among other factors, distinctive cold and warm seasons to meet their physiological requirements, progress regularly through their phenological stages, and achieve secure and sustainable production (Luedeling, 2012; Rodriguez et al., 2021). Throughout the warm temperate zone, temperate fruit crops generate essential revenue for farmers and rural communities.
Overwhelming scientific evidence indicates that recent climate change is attributable to human activity (Rai et al., 2015; Salama et al., 2021; Shukla et al., 2022). Climate change has already affected many natural ecosystems, and it threatens the stability of crop production worldwide. Anthropogenic greenhouse gas emissions have risen dramatically since the mid-20th century, driven largely by economic and population growth. The resulting increases in atmospheric concentrations of carbon dioxide, methane, and nitrous oxide are widely considered the dominant cause of increased temperatures and many extreme weather events during the last century. Future climate change is likely to cause particularly severe impacts in mid-latitude areas, which are expected to be increasingly affected by precipitation irregularities, temperature increases, and prolonged droughts (Parajuli et al., 2019; Shukla et al., 2022). These phenomena are expected to change land suitability for agricultural activities, cause variation in crop growth and development, and challenge the reliability and stability of agricultural production (Bruinsma, 2003). In some areas of the world, mainly in the tropics, increased precipitation frequency and intensity have caused devastating floods, while mid-latitudes are experiencing a decrease in the amount and frequency of precipitation (Bruinsma, 2003), leading to a rising risk of drought (Shukla et al., 2022). The instability of precipitation patterns and changes in local weather conditions are not only detrimental to crop production and quality but they are also generating social and economic uncertainties (del Pozo et al., 2019).
In addition to drought, fruit and nut crops produced in warm temperate regions are strongly threatened by temperature increases during winter, which may affect plant physiology and phenology by compromising the accumulation of winter chill, which is required for important events such as dormancy release (Luedeling, 2012; Rai et al., 2015; Rodriguez et al., 2021). The timing of dormancy release is crucial for fruit producers because, through its impact on flowering times, it determines both the amount of heat the trees can accumulate during the growing season and the risk of exposure to damaging spring frosts, which have frequently affected important growing regions in recent years (Luedeling, 2012).
Because of changing and often erratic weather patterns, agricultural production is facing increasing challenges in places where growing conditions have historically been favorable. While a number of adaptation and mitigation strategies to cope with the effects of climate change on agriculture are being explored by scientists in warm temperate regions, many of these strategies may take as long as 10 to 20 years to be implemented (Bruinsma, 2003). Promising strategies may include shifts to more suitable cultivation areas (Drogoudi et al., 2020; Fernandez et al., 2020a; del Barrio et al., 2021; Rojas et al., 2021; Noorazar et al., 2022; Meza et al., 2023), crop replacement and diversification (Valverde et al., 2015; Almagro et al., 2023; Rezgui et al., 2024), the introduction of new cultivars (Funes et al., 2016; Ruiz et al., 2019; Arenas-Castro et al., 2020; Cantin et al., 2020; Drogoudi et al., 2020), water management strategies (Fraga et al., 2020; Aguirre-Garcia et al., 2021; Gutierrez-Gamboa et al., 2022; Lulane et al., 2022; Espinoza-Meza et al., 2023; Rojano-Cruz et al., 2023), and technological adaptation (Luzio et al., 2021; Mazis et al., 2021; Rojas et al., 2021; Teker, 2023). However, as the impacts of climate change become increasingly severe, the adaptation and adoption of such strategies will require local and regional research, as well as effective governmental policies focused on the implementation of sustainable management practices and the adoption of new technologies. Consequently, it is key for scientists to identify where the current research efforts lie, and what the main knowledge gaps are.
The number of scientific publications on the effects of climate change on agricultural production has been increasing in recent decades, making it difficult for scientists to keep up with current studies and publications. Systematic reviews constitute an effective and reproducible approach to reviewing the existing literature, which allows for unbiased evidence-based conclusions (Koutsos et al., 2019). We conducted a systematic review aimed to identify the current state of the art in research, key challenges and gaps that emerge from studies of climate change effects on fruit and nut crops produced in warm temperate climates. Furthermore, along with the results obtained through the systematic review, we conducted an extended analysis beyond the scope of a regular systematic review. We identified six key research areas and grouped articles according to this classification to gauge the relative importance of these lines of research.
2 Methods
To summarize the state of knowledge, we followed the guidelines for conducting systematic reviews in agricultural sciences published by Koutsos et al. (2019), with some adjustments to fit the needs of the present work. The method we used involved six steps: 1) Scoping, 2) Planning, 3) Identification, 4) Screening, 5) Eligibility Assessment, and 6) Interpretation of Results.
The following structured questions were specified prior to the review work and further kept in mind for the development of the manuscript: 1) What are the main topics assessed in studies of climate change effects on temperate fruit and nut crop production in warm temperate climate regions? 2) What strategies have been studied to deal with climate change in temperate fruit and nut crops? and 3) Which are the most studied species in relation to climate change in warm temperate climate regions? To the best of our knowledge, no systematic reviews with the same scope and focused on the questions we addressed here have been published to date.
To carry out the literature review, we conducted a Web of Science (WoS) basic search along with a general Google Scholar search to identify studies on the effects of climate change on fruit and nut production. We identified publications that appeared particularly relevant to the objective of our study and used these studies to build a list of keywords. To identify a suitable search query for conducting this review, a series of searches were conducted in an iterative fashion between January 21st and February 10th, 2021, using the advanced search option in the WoS Core Collection database and focusing on studies that were published between 2000 and 2021. Searches were based on a three-section examination query, with sections separated by the Boolean operator “AND”. The first section was aimed at extracting publications on climate change, the second section at extracting publications on fruit and nut production, and section three at placing all publications in warm temperate climate regions. Additionally, within each section several synonyms separated by the Boolean operator “OR” were employed to maximize the capture of publications on each topic. After comparing the results obtained by several search queries, the definitive literature search was conducted on February 10th, 2021, using the following query: “TS = (((“global warming”) OR (“climate*”)) AND ((fruit*) OR (“fruit production”) OR (orchards)) AND ((“fruit growing region*”) OR (“warm temperate climate*”) OR (“temperate climate”) OR (“temperate region”) OR (“temperate”) OR (“mediterranean region”) OR (mediterran*) OR ((“wet winter*”) OR (“dry summer*”)))”, where asterisks at end of some words were used as wildcards to represent any number of letters at the end of the word. This query helped us to identify relevant publications to answer the questions addressed in this review. The search was refined to exclude reviews, proceedings, meeting abstracts, book chapters, letters, and editorial material. We complemented the original search, which was performed in early 2021, with an additional search in January 2024 to add the most recent publications.
A database containing all extracted publications was developed in Microsoft Excel for record management and examination (Figure 1). Titles, keywords and, whenever necessary, abstracts of all publications were examined to assess the potential relevance regarding the objective of this review. Records that did not fit the scope of our review were excluded from further analyses. Excluded publications focused on crops, products or activities other than temperate fruit species such as tomatoes, soybeans, wheat, cereals, mushrooms, beef, grazing, and foraging, or they featured a geographic scope outside warm temperate climate regions.
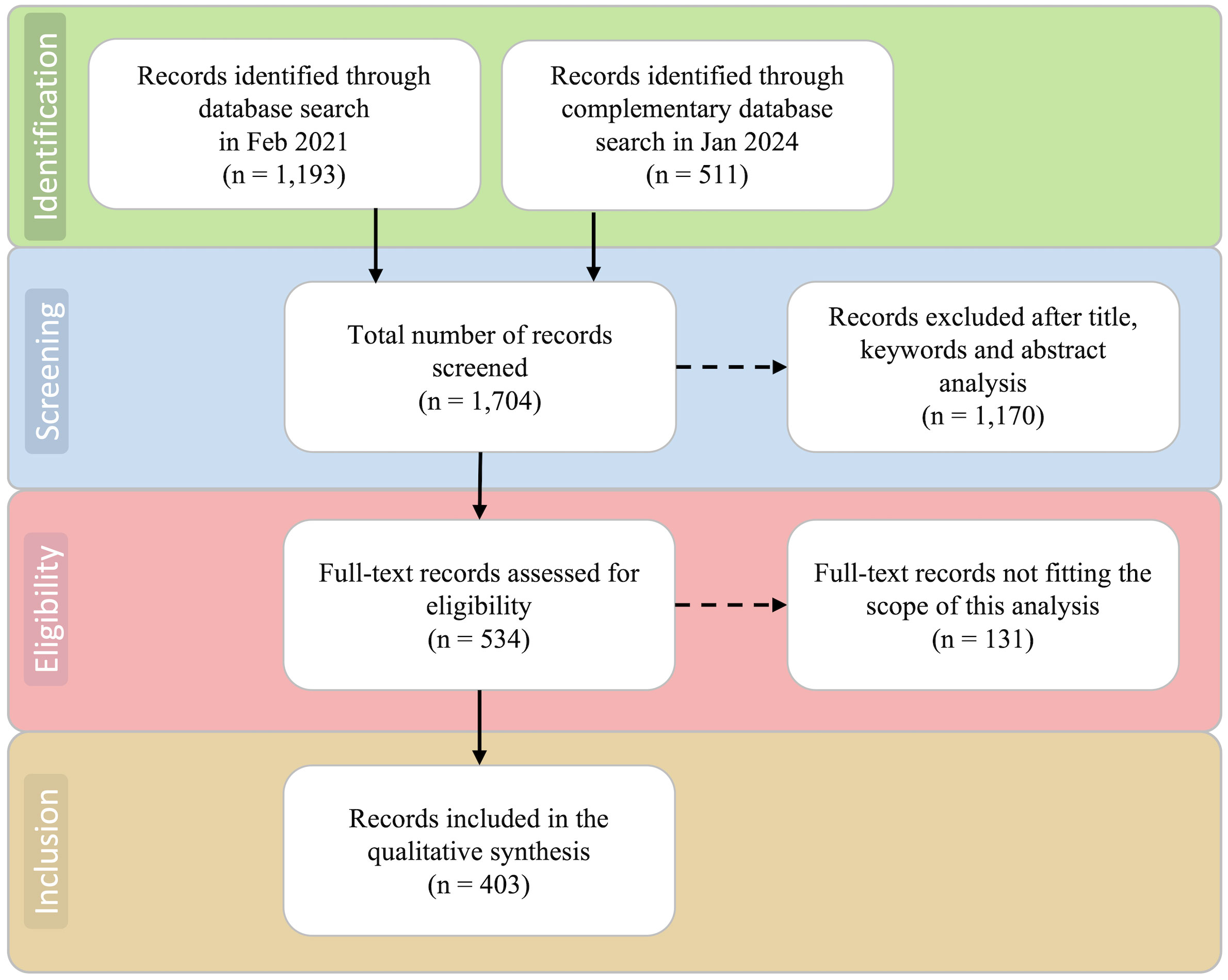
Figure 1 Schematic representation of the workflow adopted for the systematic review process, based on the PRISMA methodology (Moher et al., 2009; Koutsos et al., 2019). Dashed arrows indicate articles that were removed from the analysis. Solid arrows indicate articles retained for the next stage of the analysis.
With the definitive set of articles established after eligibility assessment, we performed a bibliometric analysis using biblioshiny, a web interface for the R package bibliometrix (Aria and Cuccurullo, 2017).We used article metadata from the Web of Science database to characterize the literature on the effects of climate change on fruit and nut crops produced in warm temperate climates for number of publications per year, country affiliation of the first author, author collaboration network, international cooperation and crops studied.
Furthermore, in an effort to better understand the results of our search, we manually characterized the literature by assigning a thematic category to each of the analyzed articles and accordingly synthesized the results within six key research areas: (1) responses to environmental conditions, (2) prediction models, (3) water management, (4) production systems, (5) sustainable agriculture, and (6) breeding & genetics. We also defined several sub-topics within each key research area to classify each article according to its research focus. However, given that an article within a major research topic may be relevant for more than one sub-topic, we do not provide a count of articles for each sub-topic (see Supplementary Table 1 for the complete list of articles classified by main research area).
3 Results
Using the definitive search query in February 2021, we identified 1,193 articles in the WoS Core Collection database. Using the same query on January 2024, we identified 511 additional articles published between 2021 and 2023. The resulting dataset consisted of 1,704 records which were further screened for eligibility. After a first evaluation, 1,170 records were excluded because they did not fit the scope of the current review. Only the remaining 534 studies assessing the effects of climate change on temperate fruit and nut production in warm temperate climates were retained.
Further manual curation allowed the detection and removal of 131 additional studies that were either conducted on crops other than temperate fruit and nut trees (e.g., avocado, coriander and pine) or not primarily focused on the effects of climate change, adaptation actions or mitigation strategies. After manual data filtering and curation, a final set of 403 articles were retained for detailed examination and used for bibliometric analyses (Figure 1; Supplementary Table 1).
3.1 Bibliometric analysis
Annual scientific productivity (i.e., the number of published articles per year) related to the effects of climate change on fruit and nut production in warm temperate climate regions has continuously increased during the last two decades (Figure 2A). No articles published in 2000, 2001, and 2003 were identified with this search. In contrast, 66 articles were identified in 2023.
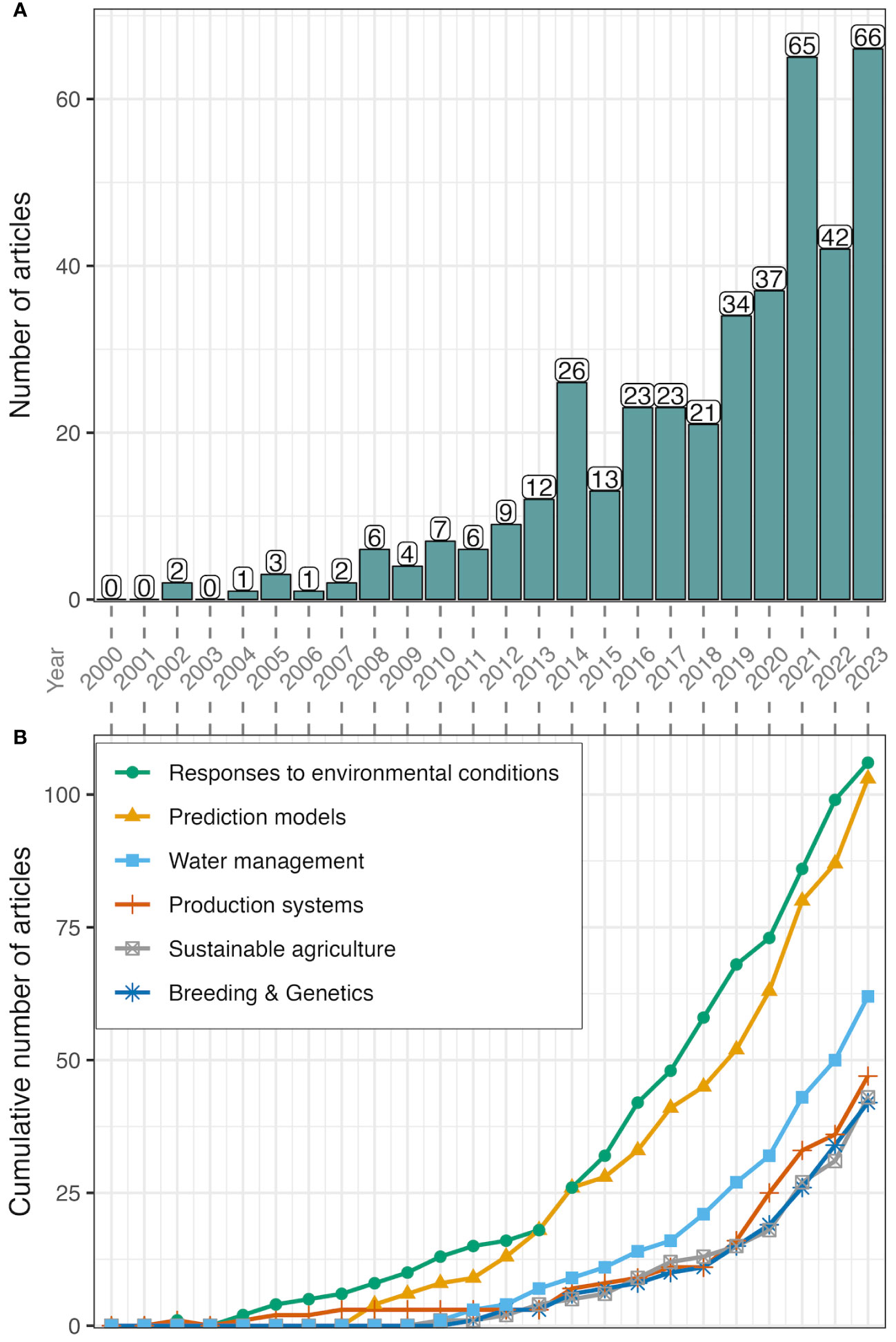
Figure 2 Scientific production by number of articles studying climate change effects on temperate fruits and nuts published by year (A) and evolution of key-topics by year (B), between 2000 and 2023. Labels of the x-axis (year) are located in between figure panels.
To visualize long-term changes in scientific productivity over time, we divided the timeframe between the years 2000 and 2023 into four six-year periods (2000-2005, 2006-2011, 2012-2017, and 2018-2023). The rising tendency observed in the yearly data became more evident when using the six-year terms, with a dramatic 44-fold difference between the first period (2000-2005: 6 articles) and the last one (2018-2023: 265 articles). Furthermore, although the magnitude of change is smaller among consecutive terms, a consistent > 2.5-fold increase demonstrates that the number of articles has increased steeply since the 2000s (2006-2011: 26 articles; 2012-2017: 106 articles).
We used the affiliations of corresponding authors as a proxy to identify regions of the world that have led scientific research on climate change and its effects on fruit and nut production in temperate regions during the last two decades. We detected a large difference between the number of articles led by authors in the northern and the southern hemispheres. Authors affiliated to institutions located north of the equator led 354 articles, representing 88% of the articles analyzed in this review. Among the countries in the northern hemisphere, Spain (90), Italy (50), USA (26) and Portugal (24) are most frequently represented (Figure 3). Conversely, only 49 articles were led by authors based in the southern hemisphere, with most of these authors affiliated with institutions in Australia (13), Chile (11), New Zealand (5) and South Africa (5). International cooperation, assessed by the number of articles with authors affiliated with institutions from more than one country, revealed that research on climate change is a highly collaborative effort, with 40% of the articles written by authors from multiple countries. Spain, Italy, USA, Portugal, Germany, and France were the countries with the highest numbers of published articles (58% of articles), with an average of 48% of them including authors affiliated to more than one country. The multiple-country publication (MCP) ratio (i.e., the proportion of articles including at least one author based in a different country from the corresponding author) shows that the Kenya (MCP ratio: 0.75), Chile (MCP ratio: 0.727), France (MCP ratio: 0.636) and Germany (MCP ratio: 0.591) have the highest proportion of articles with international collaboration (among countries with more than two publications). However, the MCP ratio may not be the best index to compare international collaboration efforts among countries because it is strongly influenced by the total number of publications. In fact, the publication outputs of the six most prolific countries (Spain, Italy, United States, Portugal, France and Germany) include 98 articles with international collaborators, compared to just 64 such publications among the remaining 38 countries (Figure 3).
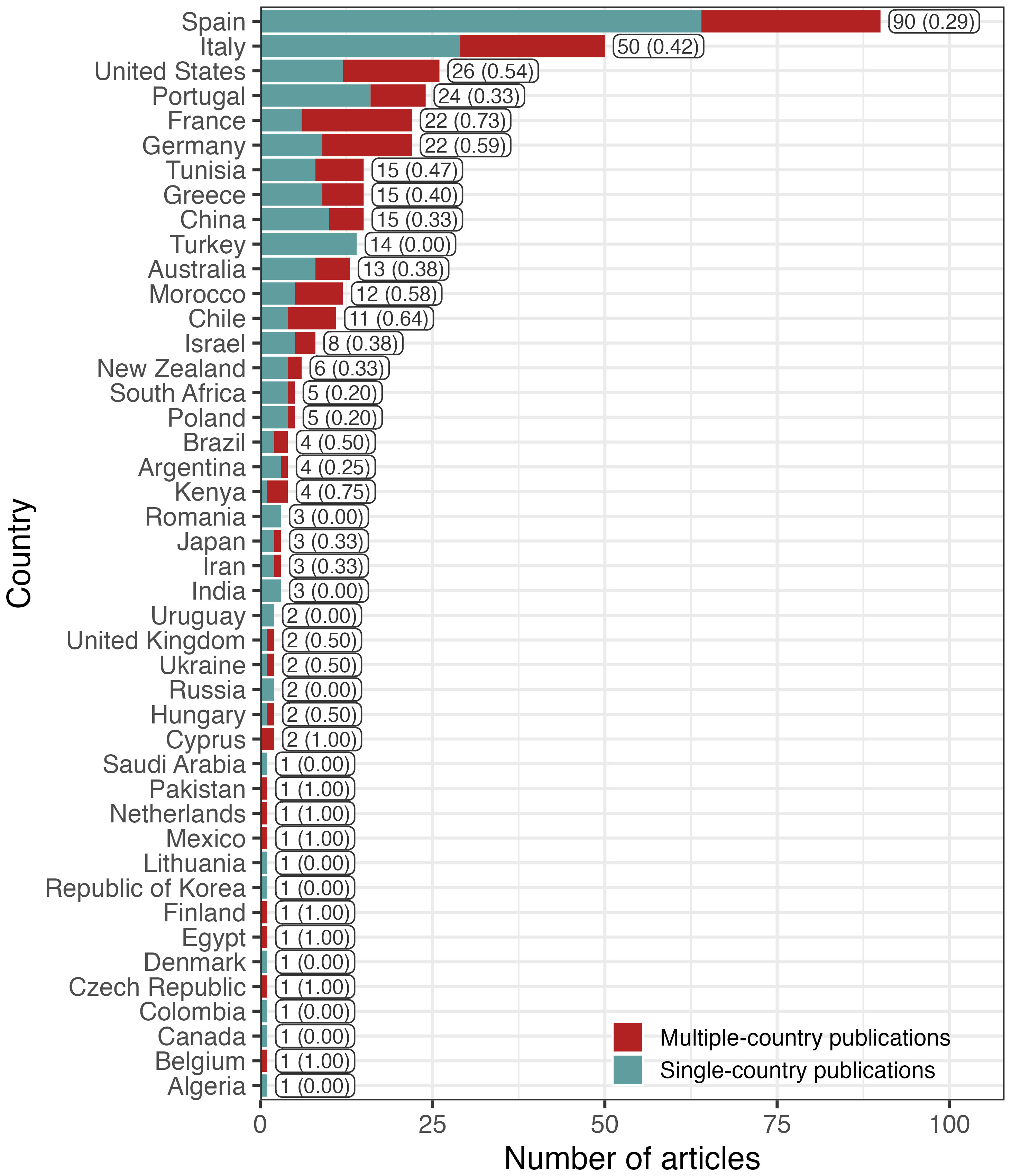
Figure 3 Total number of articles published by country, assessed by corresponding author’s country affiliation. For each country, the number of articles including authors affiliated to more than one nation (i.e., international collaboration) are shown in red and articles published by authors affiliated to a single country in green. Bar labels indicate the total number of articles per country, followed by the proportion of articles with authors affiliated to more than one country in brackets.
Based on a manual classification of articles, the ten most studied crops were olives (Olea oleracea, 92), apples (Malus domestica, 51), grapevines (Vitis vinifera, 43), almonds (Prunus dulcis, 38), cherries (Prunus avium, 33), peaches (Prunus persica, 30), citrus (24), apricots (Prunus armeniaca, 21), pistachios (Pistacia vera, 14) and pears (Pyrus communis, 12) (Figure 4). It is important to note that the number of studies focusing on olives and grapevines may be strongly influenced by the historic use of deficit irrigation strategies to manage fruit and end-product quality.
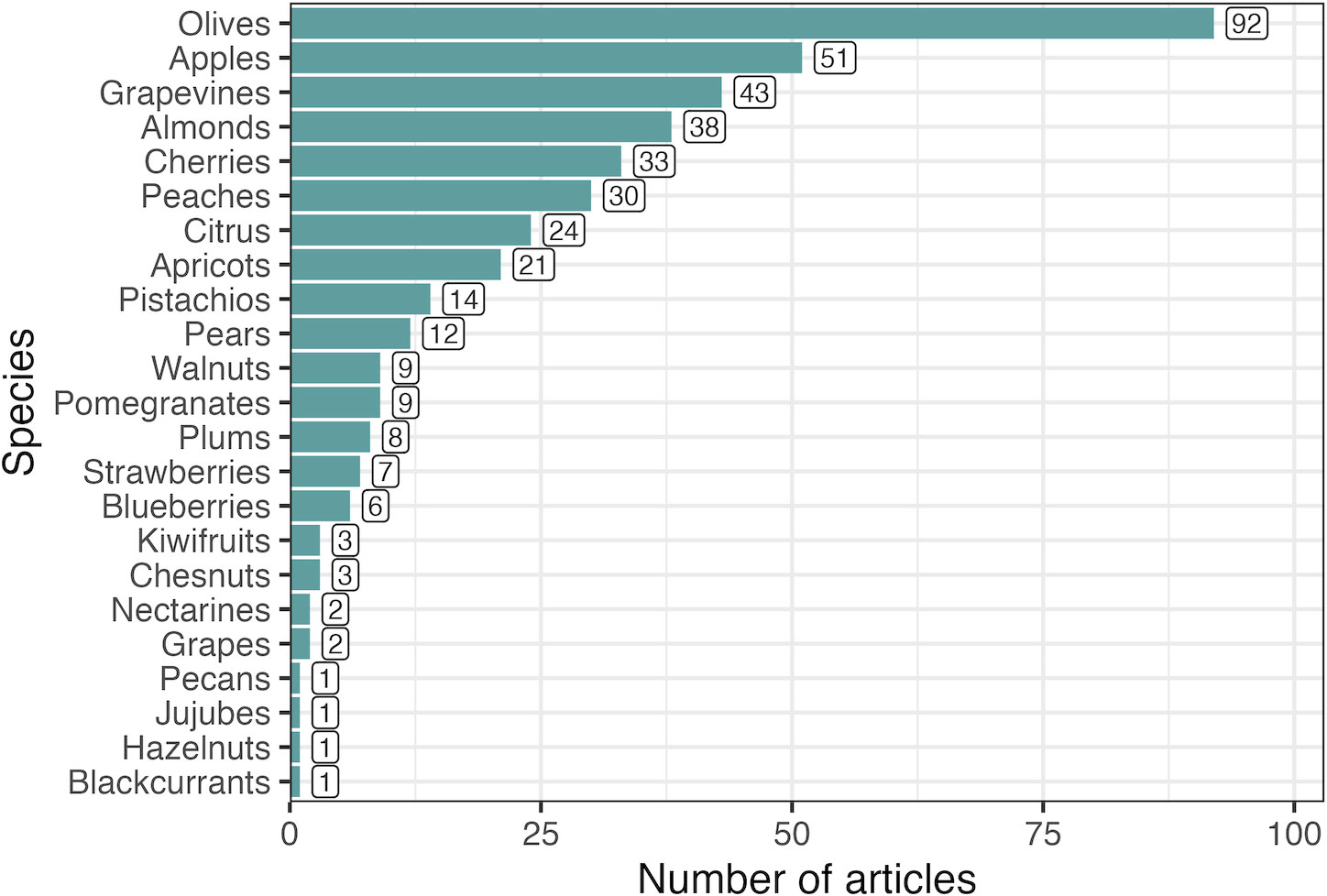
Figure 4 Most studied crops according to a manual classification of articles. Bar labels indicate the total number of articles studying a given crop. Because articles may have studied more than one species, the sum of articles per species is greater than the number of articles analyzed.
3.2 Key research topic analysis
To better understand the research focus of the literature analyzed, we manually categorized the set of 403 records used for bibliometric analyses according to six key research areas, and further divided them into several sub-topics (Figures 2B, 5). The topics and sub-topics proposed in this review are the result of a thorough manual examination. They intend to characterize the main topics studied in the articles evaluated in this review beyond the information extracted from the bibliometric analysis. It is important to note that within each major topic, many articles touched on more than one sub-topic. Although publications often provide analyses and conclusions on more than one major topic, as research in climate change is transversal by nature, here we attempted to provide an overview of each topic to characterize the state of the art (Supplementary Table 1).
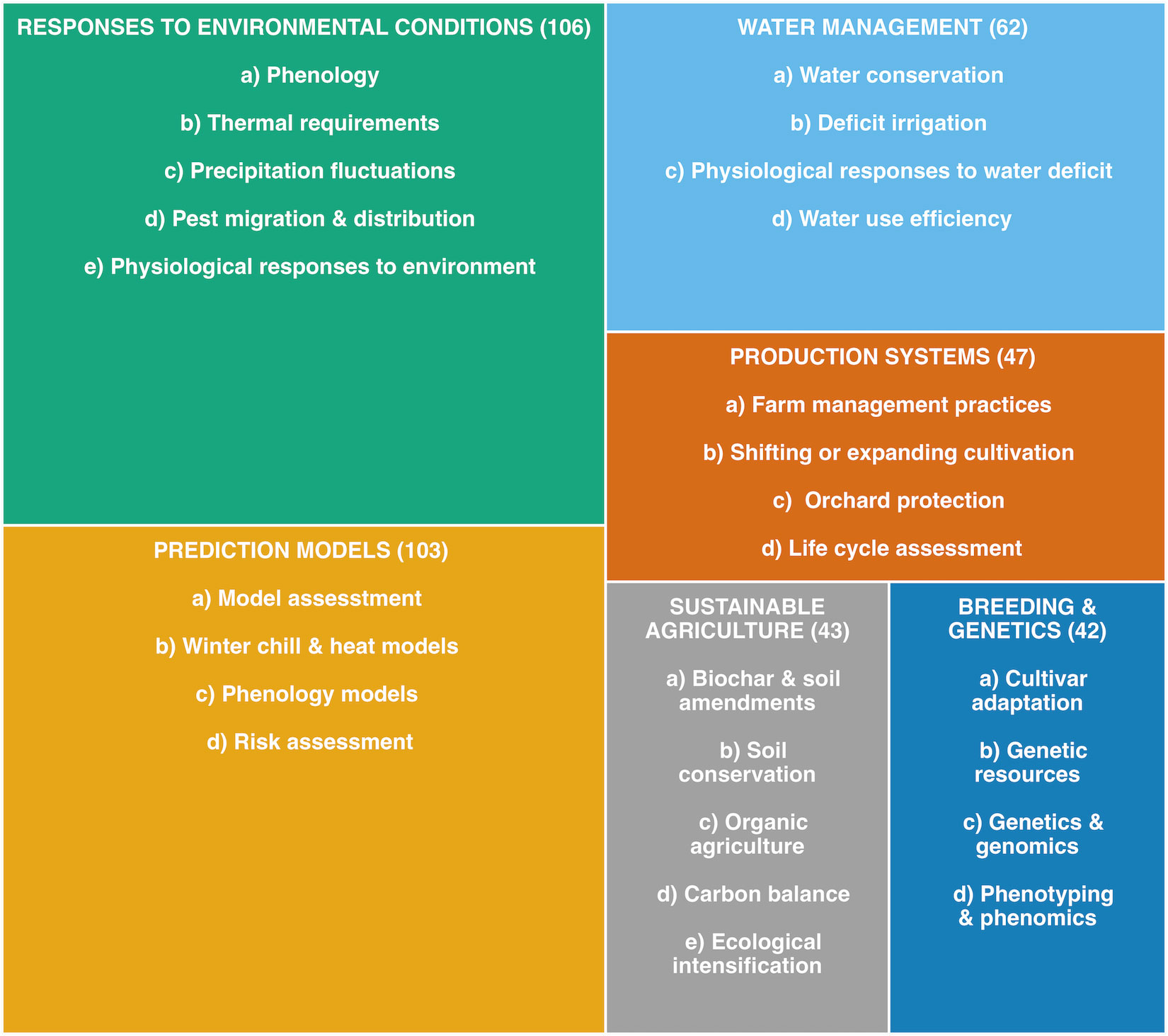
Figure 5 Key research topics and sub-topics identified based on title, abstract and full text of the 403 articles analyzed. Each of the six key research topics is listed in capital letters, and the number of studies classified under them is represented by the area of the rectangles and indicated in parentheses next to each topic. A list of sub-topics identified within each key research topic is presented below each title.
Research on plant responses to environmental conditions was the most abundant publication category, accounting for 26.3% (106 studies) of the articles included in the analysis. Within this topic, we defined five sub-topics that covered articles related to phenology, thermal requirements (chill/heat), precipitation fluctuations, pest migration and distribution, and physiological responses to the environment. With a similar number of articles, research on prediction models can be grouped into articles on model assessment, winter chill and heat models, phenology models, and risk assessment. Prediction modeling, a statistical approach to predict and forecast future events, has been a trending topic in climate change studies, as evidenced by the high number of publications detected in this review (103 studies, 25.5% of all publications). The third most abundant category grouped articles on water management, with 62 articles (representing 15.4% of the included literature) which focus on topics related to water conservation, deficit irrigation, physiological responses to water deficit, and water use efficiency. Forty-seven articles focused on research related to farm management practices, shifting or expanding cultivation, orchard protection, and life cycle assessment (LCA), representing 11.7% of the articles analyzed here. We identified 43 articles focused on topics related to the sustainable production of fruit and nut crops, accounting for 10.7% of the literature analyzed and reporting research related to the use of biochar or other soil amendments, soil conservation practices, organic agriculture, carbon balance, and ecological intensification. The sixth category, based on the number of publications, grouped articles related to breeding and genetics topics. Forty-two articles focusing on cultivar adaptation, genetic resources, genetics, genomics, and phenomics represent 10.4% of the total articles analyzed.
4 Discussion
4.1 Bibliometric analysis
Over the last two decades, the effects of climate change have become increasingly evident, with irregular weather patterns and frequent extreme climatic events impacting agriculture worldwide (Cogato et al., 2019). The steady increase in the number of publications on climate change and fruit and nut production in warm temperate climate regions reported in this analysis reflects a growing interest in research related to both a better understanding of the effects of climate anomalies on crops and the need to find strategies that allow this industry to adapt to current and future weather conditions while reducing the environmental impacts of agriculture. Perennial crops, unlike annual crops, require farmers and other stakeholders to make decisions over longer time periods (Lobos and Hancock, 2015; Ahmed et al., 2021; Meza et al., 2023). As a result, there is a need for research that can synthesize evidence on the effects of environmental factors related to climate change, as well as management conditions that are relevant to climate adaptation. This research can then inform climate change mitigation and adaptation strategies in these production systems (Ahmed et al., 2021).
Our analysis revealed a significant difference in scientific productivity between countries, based on the affiliations of the corresponding authors. Although the magnitude of the difference between hemispheres may partly be due to most countries with warm temperate climates being located north of the equator and expending more funding for this type of research, a recent study reported a similar proportion for studies on development and development policy (Amarante et al., 2022).
Authors from four countries led close to half of the articles we analyzed. Furthermore, while 40% of the articles represent international collaboration, we believe that leveraging technical skills and access to research funding and infrastructure, especially in developing nations, should be a priority (Cummings and Hoebink, 2017). For example, because breeding improved cultivars is an expensive long-term endeavor (Meza et al., 2023), developing countries may not have access to the necessary technical and financial resources to support such investment. Accordingly, both local capacity building and international collaboration, with a strong participation of local communities and considering the constraints of local production, could be an effective strategy towards the development of a more adapted agriculture that is resilient to the effects of climate change and remains sustainable over time.
4.2 Key research topic analysis
4.2.1 Responses to environmental conditions
Global warming is widely expected to increase the frequency of above-normal temperatures (Legave et al., 2015; Santos et al., 2017). Such temperatures are the main reason for the increase in heat and decrease in winter chill accumulation that have been observed in most places (Campoy et al., 2011; Luedeling, 2012; Atkinson et al., 2013; Lippmann et al., 2019). For temperate fruit and nut trees, adequate winter chill and heat accumulation are critical requirements for satisfactory commercial production (Gordo and Sanz, 2009; Cerutti et al., 2011; Luedeling et al., 2013; Darbyshire et al., 2016; Horikoshi et al., 2018; Fadon et al., 2020; Fernandez et al., 2020a; del Barrio et al., 2022). Insufficient winter chill and increasing heat during key phenological stages can affect important physiological processes, leading to non-uniform flowering (El Yaacoubi et al., 2014; Orlandi et al., 2014; Legave et al., 2015; Funes et al., 2016; Elloumi et al., 2024), delayed bud formation (Bonhomme et al., 2005; El Yaacoubi et al., 2016; Yi et al., 2020; Barba-Espin et al., 2022), abnormal bud-break (Bonhomme et al., 2005), fruit set reduction (Hedhly et al., 2007; Ruiz and Egea, 2008; Benlloch-Gonzalez et al., 2018; Egea et al., 2022), and ultimately reduced yield potential (Benmoussa et al., 2018; Moriondo et al., 2019; Mujahid et al., 2020; Fernandez et al., 2021). The literature reports a consistent decline in winter chill in all warm temperate climate regions around the world, which explains the high research interest in this topic. Since winter chill and heat accumulation are required for temperate fruit and nut crops to break dormancy, choosing appropriate cultivars for a given region is crucial to ensuring orchard productivity.
As temperature increases, prolonged drought events and variable precipitation patterns are becoming a major concern since they can cause intense drying and a reduction of irrigation water resources (Ponti et al., 2009; Gargouri et al., 2012; Ronco et al., 2017; Silva et al., 2018). Increased exposure of flowering trees to spring frost damage has also been studied, since early flowering due to increased winter temperature may expose flowers to spring frost events (Blanke and Kunz, 2009; Eccel et al., 2009; Matzneller et al., 2016; Leolini et al., 2018; Rojas et al., 2021; Yang et al., 2021; Fernandez et al., 2023). To avoid spring frost damage during the flowering period, many agricultural systems include artificial roof systems, wind machines or water sprinkler systems for protection (Ohata et al., 2017), or rely on late-blooming cultivars (Guo et al., 2019; Liu et al., 2021; Sapkota et al., 2021). Some other strategies, including the use of low-chill cultivars, dormancy avoidance, microclimate manipulation, and rest-breaking chemicals, have also been implemented to produce temperate fruit and nut crops in warm regions (Rai et al., 2015; Fernandez et al., 2023; Meza et al., 2023). Because of changing weather patterns, several studies have evaluated the capacity of crops to perform in regions that are becoming suitable for agriculture (del Barrio et al., 2021, del Barrio et al., 2022; Meza et al., 2023). However, because of the climatic variability across the world, it is imperative that further research is conducted at the local level to ensure the selection of appropriate cultivars (refer to Luedeling, 2012 for a detailed explanation).
Insect and pathogen communities are greatly affected by temperature fluctuations and climatic events. We identified several publications dedicated to studying pest migration, distribution, and adaptation, along with the risk of emerging pest threats in current and new cultivation areas due to warming weather (Gutierrez et al., 2008; Nyamukondiwa and Terblanche, 2010; Sanders et al., 2010; Sharma, 2014; Serga et al., 2015; Stephens et al., 2016; Costi et al., 2017; Gutierrez et al., 2018; Weldon et al., 2018; Qin et al., 2019; Gutierrez et al., 2021; Bogdanovich et al., 2023). Most of these articles project the future distribution of pests or changes in habitat suitability due to rising temperatures. For example, as mild winters become more frequent, effective reduction of insect populations due to cold temperature is compromised and therefore pest pressure may increase over time (Lippmann et al., 2019; Gutierrez et al., 2021). Furthermore, as spring and summer seasons become warmer, insect reproduction rates will likely increase, increasing population size, raising the pests’ ability to adapt to new scenarios, and exerting increasing pressure on host crops (Luedeling et al., 2011; Lippmann et al., 2019).
4.2.2 Prediction models
These studies have allowed the development of agroclimatic forecasts for a range of future scenarios, such as the Representative Concentration Pathways (RCPs) proposed by the IPCC, which are used to describe different climate outcomes under a range of climate forcing scenarios. For instance, winter chill and heat models, essential for quantifying winter chill and heat requirements, are fundamental for facilitating the selection of appropriate cultivars (Luedeling et al., 2009a; Luedeling et al. 2009b; Luedeling et al., 2009c; Luedeling and Gassner, 2012; Benmoussa et al., 2018; Delgado et al., 2021; Fernandez et al., 2023; Meza et al., 2023). They have been evaluated in different scenarios to determine the likelihood that a region or location can exploit new opportunities for establishing temperate orchards (Sahu et al., 2020; del Barrio et al., 2021), or to identify agricultural adaptation strategies (Cabezas et al., 2020; Meza et al., 2023).
Nevertheless, according to the reviewed literature, many of the existing models deliver questionable projections when used to predict winter chill accumulation for climate change scenarios. Models vary greatly in their sensitivity to warming (Luedeling et al., 2009b), which leads to inconsistent projections when using models across multiple locations that feature differences in climate (Fernandez et al., 2020b, Fernandez et al., 2023). Luedeling (2012) explains that several winter chill models are entirely based on empirical observations, mainly collected in the location where they were developed, leaving observed phenological variation of bloom dates across locations unexplained. Therefore, the prediction capacity of winter chill models should be questioned, and predictions should be interpreted with caution, especially given recent and projected climate change. Several authors suggest that research on prediction models and forecasting should focus on the development of updated models to ensure appropriate heat and chill quantification, helping farmers prepare for the upcoming effects of climate change (Luedeling et al., 2009b; Luedeling, 2012; Elloumi et al., 2013; Darbyshire et al., 2014; Luedeling, 2018; Campoy et al., 2019; Parkes et al., 2020; Meza et al., 2023).
Several publications included in this review focus on risk assessment analysis to comprehend the scope of climate change effects. These studies are diverse in nature, providing outcomes and guidelines for the potential risk of flood damage (Kourgialas and Karatzas, 2016), desertification and drought (Gargouri et al., 2012), water scarcity (Ronco et al., 2017; Arenas-Castro et al., 2020), and yield potential (Fraga et al., 2020), among other factors. Having the ability to predict the future through models greatly enhances our ability to study and understand prospective climate outcomes, and to develop adequate responses to mitigate or adapt to the predicted conditions. Risk assessments have also been used to evaluate the likelihood of a cultivar meeting its winter chill requirements (Measham et al., 2014; Darbyshire et al., 2016; Fernandez et al., 2020a), and to provide relevant data through a platform targeting growers and researchers to support strategic orchard management decisions (Pathak et al., 2018; Fernandez et al., 2022). Accurate prediction models can provide crucial insights to policymakers and stakeholders to develop and implement adaptation strategies that help secure the sustainability of the fruit industry under future scenarios.
4.2.3 Water management
Water scarcity, aggravated by the effects of climate change, is predicted to worsen due to more severe and frequent drought events in warm temperate climate regions (Ronco et al., 2017; Galindo et al., 2018; Pedrero et al., 2020). This will decrease land suitability for fruit crops in many regions, especially in arid and semi-arid areas where rainfall is becoming less intense and frequent (Ronchail et al., 2014; Galindo et al., 2018; Araya-Osses et al., 2020; El-Otmani et al., 2020; Meza et al., 2023). Increasing annual mean temperature due to climate change also threatens the availability of freshwater. Along with reductions in water availability, higher temperatures increase evapotranspiration and crop water demand (Rai et al., 2015; del Pozo et al., 2019; Arenas-Castro et al., 2020; Fraga et al., 2020). The aforementioned conditions may explain the strong focus on water-saving strategies, such as water deficit irrigation management, that we observed in our analysis.
Deficit irrigation has historically been the main strategy used in olive trees and grapevine to manage fruit quality (Fernandes de Oliveira et al., 2013; Galindo et al., 2018; Araujo et al., 2019; Buesa et al., 2019; Kokkotos et al., 2020; Siakou et al., 2021; Ibba et al., 2023; Ferrara et al., 2024), and it is becoming a popular strategy in other crops, including mandarins (El-Otmani et al., 2020), pomegranates (Galindo et al., 2018; Adiba et al., 2022), apples (Panzacchi et al., 2012; Kendall et al., 2022), almonds (Gutierrez-Gordillo et al., 2020; Barreales et al., 2023), peaches (Ruiz-Sánchez et al., 2018), apricots (Ezzat et al., 2021), and pistachios (Marino et al., 2018). In brief, the idea is to reduce the amount of water provided to the crop during the growing season, improving marketable yield per unit of water and end-product quality, rather than achieving maximum yields (Ronco et al., 2017; Galindo et al., 2018; El-Otmani et al., 2020). However, some physiological stages such as blooming and fruit or nut filling are very sensitive to water deficit. Hence, deficit irrigation strategies need to be tailored for the specific needs of each crop, to maximize water savings without compromising fruit quality and yield (Galindo et al., 2018; Ruiz-Sánchez et al., 2018). A deficit irrigation strategy that is carefully adjusted to crop needs during all phenological stages can maximize water savings, without impacting fruit size and quality (Ruiz-Sánchez et al., 2018). Galindo et al. (2018) provide a complete review of water deficit strategies, proposing adoption of crops that are able to withstand low water supply, and highlighting the need for research on the risk of soil salinization, which may result from such irrigation strategies.
Two other strategies for water-savings that were mentioned in the reviewed literature are the use of desalinated water (Martin-Gorriz et al., 2014; Romero-Trigueros et al., 2021) and wastewater for irrigation purposes (Moretti et al., 2019; Kokkotos et al., 2020). Nonetheless, Martin-Gorriz et al. (2014) state that, while possibly conferring some adaptation benefits to fruit producers, using desalinated water may also accelerate global warming, since water desalination is an energy-intensive process. However, other authors propose that as water desalination is becoming cheaper and more sustainable, more research on the safe use of desalinated water in agriculture is needed (Aldaya et al., 2019). Besides desalinated water, wastewater is a plausible source for supplementing irrigation water in regions where drought is limiting food production (Moretti et al., 2019; Pedrero et al., 2020). Wastewater may come from different sources such as municipal effluents, agriculture, animal production and industrial processes (Pedrero et al., 2020). Nevertheless, in addition to the treatment required to safely use wastewater for irrigation in agriculture (Meli et al., 2002; Pedrero et al., 2020), some parameters such as salinity, sodicity, metal and trace elements, and organic materials may need to be closely monitored and controlled to ensure the safety of using such water resources for crop irrigation (Moretti et al., 2019; Pedrero et al., 2020).
Since water is essential for basic physiological processes, several studies have focused on the physiological responses of trees to water stress (Panzacchi et al., 2012; Zapata-Sierra and Manzano-Agugliaro, 2017; Torres et al., 2018; Gutierrez-Gordillo et al., 2020; Adiba et al., 2021). Examples of such processes and phenomena whose responses to water stress have received scientific attention are berry composition in grapevines (Fernandes de Oliveira et al., 2013; Permanhani et al., 2016; Buesa et al., 2019), anthocyanin levels in grapes (Fernandes de Oliveira et al., 2013), fruit quality in citrus and pistachios (Garcia-Tejero et al., 2011; Marino et al., 2018), phenolic composition in sweet cherries (Blanco et al., 2020), biomass in olives (Viola et al., 2012, Viola et al., 2014; Pierantozzi et al., 2020) and yield increase or decrease in grapes, olives and pistachios (Garcia-Tejero et al., 2011; Viola et al., 2012; Permanhani et al., 2016; Blanco et al., 2020; Pierantozzi et al., 2020). Most of these studies were aimed at increasing fruit quality and crop sustainability under water scarcity.
In some countries of the warm temperate climate region, governments are promoting or investing in irrigation infrastructure and encouraging farmers to adopt new technologies for sustainable irrigation (Roco et al., 2014; Galindo et al., 2018). However, such adaptation actions can be difficult to take. In Chile, for instance, farm-level adaptation is lagging behind scientific knowledge and government policies, mainly because of farmers’ highly variable socio-economic conditions and the high level of investment required to establish such infrastructure (Roco et al., 2014). Nonetheless, farmers’ ability to strategically manage water resources and to attune water availability to the needs of each specific crop and cultivar may make a difference in preserving freshwater for future generations.
4.2.4 Production systems
Weather instabilities along with extreme climatic events have forced growers to look for agricultural practices that can help them adapt to the changing climatic regime. Rest-breaking chemicals have often been used to compensate for the lack of chill accumulation caused by increased temperatures (Gariglio et al., 2012; Ghrab and Ben Mimoun, 2014). As an alternative to chemical treatments, Mujahid et al. (2020) proposed using a cold plasma treatment to break dormancy in grapes. Beyond chill and dormancy control, optimizing nutrient use efficiency (Swarts et al., 2016), irrigation scheduling and harvest timing (Paltineanu and Chitu, 2020) are other farm management practices mentioned in the literature that can be used to reduce greenhouse gas emissions and facilitate climate change mitigation.
Microclimate management, such as water supply management or hail and shade nets, as well as soil management, such as tilling or mulching, can be effectively used in production systems to help reduce agriculture’s pressure on the environment (Houston et al., 2018; Campi et al., 2020). Protective covers and canopy management techniques are often used to mitigate the effects of climate change and have allowed for the expansion of agricultural production to new production areas. For instance, the use of polyethylene covers was evaluated in sweet cherry orchards in Chile, where such protective measures may provide protection from rain, hailstorms, and extreme cold temperatures as a strategy to expand production to new potential growing regions (Blanco et al., 2021; Rojas et al., 2021).
Along the same lines, another promising strategy identified in the literature to reduce the risk of insufficient winter chill accumulation or escape spring frost damage and desertification is the redistribution, shifting or expansion of cultivation to regions that may become favorable for fruit crop production, leading to the reconfiguration of agricultural landscapes (Ponti et al., 2009; Luedeling et al., 2009a; Dag et al., 2014; Tanasijevic et al., 2014; Thomson et al., 2014; Ohata et al., 2017; Rana et al., 2017; Hepaksoy et al., 2018; Leolini et al., 2018; Guo et al., 2019; Ben Rouina et al., 2020; Sahu et al., 2020; del Barrio et al., 2021; Rodriguez et al., 2021; Meza et al., 2023). The main limitation to most of these studies, however, is that they are solely based on the configuration of bioclimatic conditions, without considering local agricultural land use distribution, competition between land uses, and the adaptive capacity of crops (Houston et al., 2018; Sahu et al., 2020; del Barrio et al., 2021). A study in Argentinian Patagonia concluded that the increasing heat availability in the south of the southern hemisphere presents an opportunity for fruit and nut growers since new species and cultivars with temperate-climate requirements can be introduced into new regions outside their traditional ranges (del Barrio et al., 2021, del Barrio et al., 2022). Other studies evaluated the performance of olives in hot desertic areas to establish their yield potential and olive oil quality for future production (Dag et al., 2014; Ben Rouina et al., 2020).
Different strategies have also been proposed to protect crops from rising temperature and diminish sunburn damage. Excess heat and the additional water stress that it causes, can lead to a decrease in growth, leaf function, productivity, and fruit quality. Therefore, it is important to ensure that adequate measures are in place to mitigate the impact of these factors on plant health and overall performance. White kaolin powder sprays, clay, calcium carbonate and wax emulsion sprays have been used to reduce radiation load by increasing canopy albedo (Rosati et al., 2007; Houston et al., 2018; Meza et al., 2023; Teker, 2023). Other methods proposed to reduce radiation include leaf canopy management to keep the fruit shaded (Feng et al., 2018; Garrido et al., 2018; Martínez-Lüscher et al., 2020; Meza et al., 2023) and the installation of shading nets covering orchards and vineyards (Lobos et al., 2012; Feng et al., 2018; Mupambi et al., 2018). Besides methods oriented to reduce radiation, studies support the use of overhead irrigation misting systems to avoid heat stress in mid-summer (Kliewer and Schultz, 1973; Feng et al., 2018; Martínez-Lüscher et al., 2020).
4.2.5 Sustainable agriculture
According to the Intergovernmental Panel on Climate Change (Shukla et al., 2022), the agriculture, forestry, and other land uses (AFOLU) sector was responsible for 13 - 21% of direct anthropogenic greenhouse gas (GHG) emissions between 2010 and 2019. On the other hand, agriculture is also responsible for considerable carbon sequestration through soil and plant activity, which contributes to mitigating climate change (Aguilera et al., 2015; Mohamad et al., 2016; Almagro et al., 2017; Shukla et al., 2022).
Intensive agricultural farming systems require a lot of energy for food production (Mazis et al., 2021). Conventional farming systems coupled with climate change often lead to the reduction of soil organic matter, soil erosion, desertification, and degradation of water resources (Michalopoulos et al., 2020; Niu et al., 2021), leading to less carbon storage in those systems. However, research in olive orchards supports that intensification can improve carbon sequestration and soil quality (Mairech et al., 2020, Mairech et al., 2021; Taguas et al., 2021). Although sustainable agricultural practices have been applied for a long time, as the effects of climate change become more evident, alternative farming practices have recently gained new prominence due to conducive policies and increased consumer demand for fruits and nuts with low environmental impact.
Sustainable practices, such as minimum or no tillage (Cook, 2006; Rodriguez-Pleguezuelo et al., 2017), weed mowing (Martin-Gorriz et al., 2020), cover cropping (Marquez-Garcia et al., 2013; Wolf et al., 2017), incorporation of biochar and organic soil amendments (Stavi, 2013; Baronti et al., 2014; Walkiewicz et al., 2020), biomass accumulation and crop diversification (Martin-Gorriz et al., 2020; Michalopoulos et al., 2020), are potentially beneficial measures to offset anthropogenic greenhouse gas emissions (Stavi, 2013) and provide efficient mechanisms against soil degradation and desertification (Martin-Gorriz et al., 2020). However, none of these practices alone consistently leads to positive effects on ecosystems (Kleijn et al., 2019a). More studies on sustainable practices may be needed at the regional level to help reduce knowledge gaps related to local farming practices and to help farmers make informed decisions.
Organic agriculture has been proposed and evaluated as an adaptation strategy to help reduce the impacts of climate change (Mohamad et al., 2016). Granatstein et al. (2016) reported that organic agriculture has grown by 109% in Europe and North America for organic temperate fruit trees between 2008 and 2013. Some benefits derived from organic farm management include increased carbon sequestration, reduced erosion, soil fertility restoration and biomass accumulation (Martin-Gorriz et al., 2020). However, Mohamad et al. (2016) found that the use of manure for fertilization in organic agriculture increased GHG emissions, even though an increase in soil carbon content compensated, at least partially, for the negative effect of these emissions.
Ecological intensification is based on the assumption that the delivery of ecosystem services is suboptimal in high-input agricultural systems (Bommarco et al., 2013). This production strategy has been proposed as an alternative to maximize productivity while minimizing potential negative environmental effects, by harnessing ecosystem services to complement or substitute external inputs, such as synthetic fertilizers and agrochemicals (Bommarco et al., 2013; Kleijn et al., 2019b). Despite the use of wildflower strips having become a popular strategy in temperate orchard systems, their implementation in Mediterranean orchard systems remains understudied (Mockford et al., 2023). In a recent study, Mockford et al. (2023) evaluated the suitability of 12 native perennial species for wildflower strips in commercial citrus orchards over a three-year period, reporting increasing plant species richness and greater availability of resources expected to support natural enemies. Agroforestry is a model of ecological intensification that has the potential to provide multiple ecosystem services and contribute to biodiversity conservation in agricultural landscapes (Torralba et al., 2016; Panozzo et al., 2022). These systems have been associated with improvements in crop growth and yield, particularly in tropical and subtropical regions (Jose, 2009; Tscharntke et al., 2011; Mbow et al., 2014). In the Mediterranean area, where the risk of yield losses due to climate issues is increasing, the impact of trees on the microclimate and edaphic environment might be beneficial for understory crops (Panozzo et al., 2022). Although agroforestry has a long tradition in the Mediterranean region dating back to pre-Roman times, due to agricultural intensification, traditional agroforestry systems have not been implemented in the last few decades in a large part of this area (Panozzo et al., 2022; Rezgui et al., 2024). We identified four studies published recently focusing on the implementation of agroforestry systems to enhance farm sustainability and diversification (Bateni et al., 2021; Zahoor et al., 2021; Panozzo et al., 2022; Rezgui et al., 2024).
Carbon sequestration has also been studied as a mitigation measure to restore degraded land. Temperate fruit orchards and vineyards are currently receiving much attention for their potential to act as carbon sinks to help raise soil organic matter contents (Phani Kumar et al., 2010; Scandellari et al., 2016; Mairech et al., 2020; Mairech et al., 2021; Taguas et al., 2021). Montanaro et al. (2017) studied carbon budgets in peaches with the purpose of establishing the best soil management method to improve carbon sequestration and raise soil organic carbon content. Overall, the research we reviewed indicates that advances towards sustainable and climate-smart fruit production can be achieved through a range of practices, including improving soil management, incorporating crop residues and reducing tillage.
It is important to highlight that major advances towards sustainable agricultural production across the globe seem unlikely without changes in policies, economic incentives and farm adaptation (Shukla et al., 2022). Such measures need to consider the costs and benefits for farmers, including the impact on crop yield stability. Therefore, most authors agree that adequate strategies for sustainable farm management need to be implemented at local level in order to reduce GHG emissions. Sustainable farming practices that combine elements of conventional and organic practices may hold promise for reducing the negative impact of agricultural practices on the environment (Ceccanti et al., 2020).
4.2.6 Breeding and genetics
Genetic improvement is a long-term strategy that involves the development of new, adapted cultivars that are able to withstand the adversities of climate change (Iwata et al., 2016; Houston et al., 2018). To improve knowledge and enhance the capacity to react to changing climate conditions, breeders should have access to genetic diversity and genetic resources that thrive under marginal growing conditions (Hoisington et al., 1999; Brummer et al., 2011; Lippmann et al., 2019; Galluzzi et al., 2020; Letelier et al., 2020). Consequently, access to genetic resources and their evaluation for climate adaptation was identified as a topic of interest within the scientific community. Galluzzi et al. (2020) conducted a survey on the role of genetic resources in breeding for climate change in developing countries, finding a prevailing tendency among respondents to use advanced or elite germplasm as a source of genes to breed for drought tolerance. However, genetic diversity within elite germplasm may be low, and alleles related with adaptation to non-optimal growing conditions may have been lost during crop domestication and breeding. Breeders have started looking for genetic sources outside of modern cultivars in search of traits that may enable crops to tolerate prevailing or expected environmental conditions. Wild and domesticated accessions stored in gene banks around the world may contain genes related to crop tolerance to increased annual temperatures, reduced winter chill accumulation, reduced annual precipitation, prolonged drought events, and increased pest pressure that are not present in elite materials. Perez et al. (2020) evaluated old peach cultivars on the Canary Island La Palma, to look for germplasm adapted to warmer conditions, and Carvalho et al. (2017) evaluated ten traditional Portuguese grapevine varieties to identify genes associated with tolerance to abiotic stresses. In olive, a crop that is highly adapted to dry conditions, a collection of wild crop relatives was evaluated with microsatellite markers to determine suitable genotypes to be used as rootstocks to improve olive productivity (Diaz-Rueda et al., 2020a). Nevertheless, according to Galluzzi et al. (2020), the lack of knowledge on genetic resources and cultivar diversity in several species limits their use for accessing important traits for adaptation to climate change conditions.
Genetic resources in the form of cultivated crops are threatened by global warming, which may reduce the fitness of many wild species in their natural habitats (Lippmann et al., 2019). The loss of natural habitat and crop genetic diversity and the disappearance of native germplasm signify setbacks for breeding efforts for climate adaptation. Access to a wide range of genetic diversity is critical for the success of plant breeding agendas (Hoisington et al., 1999), highlighting the importance of strengthening policies to incentivize germplasm collection and conservation.
Although natural populations and germplasm collections may harbor genes that can confer adaptation benefits to commercial crops (Lobos and Hancock, 2015; Perez et al., 2020; Diaz-Rueda et al., 2020b), to facilitate their rapid adoption by breeding programs, genomic regions associated with traits of interest need to be mapped and characterized. Novel genomic technologies developed in recent years have provided new gene mapping techniques and allowed the development of molecular markers that can accelerate the efficient deployment of beneficial alleles to new cultivars (Lobos and Hancock, 2015; Iwata et al., 2016; Whitaker et al., 2020). For instance, because chilling requirements are becoming a limiting factor for cultivar adaptation in warming environments, scientists are working on identifying genes associated with bud dormancy and flowering time in temperate crops to elucidate the genetic control of these processes and develop new cultivars adapted to different climatic conditions (Castede et al., 2014; Romeu et al., 2014; Allard et al., 2016; Prudencio et al., 2018; Cantin et al., 2020; Prudencio et al., 2021; Zhang et al., 2022). New technologies have also enabled the identification of the genetic regions controlling traits such as flowering time (Ruiz et al., 2019; Branchereau et al., 2023) and bud dormancy (Allard et al., 2016; Prudencio et al., 2018, Prudencio et al., 2021), as well as traits related to temperature requirements for endodormancy release (Romeu et al., 2014). The identification of regions harboring genes related with the response of trees to environmental cues has facilitated the efficient use of molecular markers to reliably select genotypes that are better suited to particular climatic conditions.
As mentioned before, new pathogens will probably migrate to temperate regions, posing new challenges for disease management. In consequence, resistance to pests and diseases is one of the main objectives for breeding programs, and it will continue to play an important role along with the improvement of other phenotypic traits to overcome the challenges of climate change (Brummer et al., 2011; Lippmann et al., 2019). For a cultivar to be successful, adaptation and performance must be evaluated in multi-environment trials to assess the effects of genetic and environmental factors, as well as the effects of interactions between these factors on crop quality and productivity (Navas-Lopez et al., 2019; Farsi et al., 2023). Such trials are instrumental for identifying cultivars that produce reliably across environments or to select the best performers for specific conditions. In this context, the role of breeders is to identify the individuals with the highest breeding value given the local environment. Hence, local research organizations and universities, governments and private companies need to invest in local breeding programs to take timely actions in response to the needs of local environments and markets (Brummer et al., 2011; Galluzzi et al., 2020).
5 Conclusions and future direction
With the rapid expansion of climate change-related literature, systematic analyses can support researchers aiming to identify aspects that are well understood, topics that have remained unexplored and urgent questions that need to be addressed in the future.
Despite the possibility that we may have missed some pertinent articles due to methodological limitations, we believe that our key research topic analysis provides a good overview of the state of knowledge and constitutes a valuable resource that can help researchers from different disciplines identify critical knowledge gaps. Based on our systematic literature review, we find that several prominent topics have not or insufficiently been covered in the existing literature:
i. The recent rapid development and declining costs of molecular tools and computational capabilities have provided new opportunities to accelerate breeding of new cultivars adapted to extreme conditions. New approaches include high-throughput phenotyping and genotyping, genomic selection and predictive breeding driven by artificial intelligence. Efforts are still needed to adjust such technologies to the particular biological context of fruit and nut trees.
ii. The possible medium- or even long-term impacts of climate change on dormancy and phenology of temperate fruit trees remain unclear. To our knowledge, most studies have focused on immediate impacts, with little attention to tree responses during subsequent growing seasons. Knowledge about the trees’ ability to reset after unusually warm or dry seasons (as well as on the mechanisms involved) can greatly improve our understanding of factors that regulate dormancy during winter. Similar to developing new cultivars, such advances may also present opportunities for the development of effective adaptation strategies.
iii. After decades of stagnation, the validation, development and application of dormancy models has picked up speed during the time period we evaluated. Even though this work has led to some promising new avenues in modeling tree dormancy, it has also exposed glaring knowledge gaps. Prominent gaps are related to possible species- or cultivar-specific differences in chill (and heat) responses, to the precise nature of chill and heat accumulation dynamics, and to the validity and reliability of the models that are currently applied by scientists and practitioners.
iv. What remains conspicuously absent are dormancy models that are based on the current state of knowledge across the whole range of disciplines that have focused on dormancy research. Even though a comprehensive conceptual model of tree dormancy has been proposed (Fadon et al., 2020), this model still appears far from being turned into a computable model that could be used for predictions and for guiding further research on dormancy. Possibly, knowledge on tree dormancy is still too fragmented or the dormancy release process may be too complex to be reliably modeled at this point in time, but we remain hopeful that the coming decade(s) will deliver some progress in this space.
v. While Mediterranean climate regions have traditionally been ideal for temperate fruit crops, climate change related issues such as insufficient water availability for irrigation or deficient chill accumulation threaten production in these areas. The implementation of protective cover technologies has allowed for an expansion of production to cooler areas where drought and chill accumulation are not yet limiting production. However, these adaptation strategies cause significant microclimate variation with potential impacts on physiological processes and tree phenology. As the use of protective covers becomes more frequent, further research on the effects of such practices on tree nutrient and water demand is needed for designing management strategies that optimize yield and quality.
vi. Agroecological management can also be evaluated as an effective strategy to increase climate resilience. Although some studies found in this review addressed sustainable management practices such as non or minimum tillage, green manure, and soil amendments, the agroecological perspective involves a paradigm shift that considers the ecological equilibrium of the agroecosystem as a management strategy, focused on decreasing the dependence on external inputs. System that are guided by agroecological principles have been claimed to be comparatively resilient to climatic shocks and stresses, among other benefits (Altieri et al., 2015). More research focused on evaluating the effects of integrated agroecological management rather than assessing the effects of individual sustainable management practices is expected to support the transition towards systems that can adapt to current and future challenges.
Growing awareness of the effects that climate change may have on the future of our planet has triggered various local and international initiatives aimed at slowing down and hopefully reversing the buildup of greenhouse gases in the atmosphere. Here we report a diverse range of strategies focused on both mitigating the environmental impacts of fruit and nut production, and on adapting to current challenges stemming from changing weather patterns and extreme weather events that have become increasingly frequent and intense. However, the development and implementation of a set of strategies that secure the long-term sustainability of agricultural production and support farmers in adapting to future weather patterns may take a long time and require a multidisciplinary global effort. With varying vulnerability to climate change and diverse social and economic realities in different regions, policy changes along with technological development (e.g., breeding new cultivars, more efficient irrigation systems and water management and sustainable agricultural practices) need to be carefully crafted to meet local needs.
Finally, we strongly believe that global collaborative efforts that engage all actors of the production chain, including growers, distributors, retailers, and consumers, may optimize the chances of success in adapting the production of temperate fruit and nuts to the challenging future that lies ahead.
Data availability statement
The original contributions presented in the study are included in the article/Supplementary Material. Further inquiries can be directed to the corresponding author.
Author contributions
JO-M: Conceptualization, Data curation, Formal analysis, Investigation, Methodology, Visualization, Writing – original draft, Writing – review & editing. EF: Visualization, Writing – review & editing. LV: Conceptualization, Writing – review & editing. AR: Conceptualization, Funding acquisition, Writing – review & editing. EL: Visualization, Writing – review & editing. NC: Conceptualization, Formal analysis, Funding acquisition, Methodology, Supervision, Validation, Visualization, Writing – original draft, Writing – review & editing.
Funding
The author(s) declare financial support was received for the research, authorship, and/or publication of this article. This research was supported by the Chilean Ministry of Education, project FRO1795 “Fruticultura Sin Fronteras” and by the National Research and Development Agency (ANID), Chile, project PAI77190085. NC also acknowledges funding received from Universidad de La Frontera, project DI21-0104. We thank the Partnership for Research and Innovation in the Mediterranean Area (PRIMA), a program supported under H2020, the European Union’s Framework program for research and innovation, for partly supporting this research within the AdaMedOr project (grant number 01DH20012 of the German Federal Ministry of Education and Research).
Conflict of interest
The authors declare that the research was conducted in the absence of any commercial or financial relationships that could be construed as a potential conflict of interest.
Publisher’s note
All claims expressed in this article are solely those of the authors and do not necessarily represent those of their affiliated organizations, or those of the publisher, the editors and the reviewers. Any product that may be evaluated in this article, or claim that may be made by its manufacturer, is not guaranteed or endorsed by the publisher.
Supplementary material
The Supplementary Material for this article can be found online at: https://www.frontiersin.org/articles/10.3389/fpls.2024.1352169/full#supplementary-material
References
Adiba, A., Hssaini, L., Haddioui, A., Hamdani, A., Razouk, R. (2022). Biochemical properties of pomegranate (Punica granatum L.) juice as influenced by severe water stress. Sci. Hortic. 304, 111286. doi: 10.1016/j.scienta.2022.111286
Adiba, A., Razouk, R., Charafi, J., Haddioui, A., Hamdani, A. (2021). Assessment of water stress tolerance in eleven pomegranate cultivars based on agronomic traits. Agric. Water Manage. 243, 106419. doi: 10.1016/j.agwat.2020.106419
Aguilera, E., Guzmán, G., Alonso, A. (2015). Greenhouse gas emissions from conventional and organic cropping systems in Spain. II. Fruit tree orchards. Agron. Sustain. Dev. 35, 725–737. doi: 10.1007/s13593-014-0265-y
Aguirre-Garcia, S.-D., Aranda-Barranco, S., Nieto, H., Serrano-Ortiz, P., Sanchez-Canete, E.-P., Guerrero-Rascado, J.-L. (2021). Modelling actual evapotranspiration using a two source energy balance model with Sentinel imagery in herbaceous-free and herbaceous-cover Mediterranean olive orchards. Agric. For. Meteorol. 311, 108692. doi: 10.1016/j.agrformet.2021.108692
Ahmed, S., Brinkley, S., Smith, E., Sela, A., Theisen, M., Thibodeau, C., et al. (2021). Climate change and coffee quality: systematic review on the effects of environmental and management variation on secondary metabolites and sensory attributes of coffea arabica and coffea canephora. Front. Plant Sci. 12. doi: 10.3389/fpls.2021.708013
Aldaya, M. M., Custodio, E., Llamas, R., Fernández, M. F., García, J. (2019). and ródenas, M An academic analysis with recommendations for water management and planning at the basin scale: A review of water planning in the Segura River Basin. Á.Sci. Total Environ. 662, 755–768. doi: 10.1016/j.scitotenv.2019.01.266
Allard, A., Bink, M. C. A. M., Martinez, S., Kelner, J.-J., Legave, J.-M., di Guardo, M., et al. (2016). Detecting QTLs and putative candidate genes involved in budbreak and flowering time in an apple multiparental population. J. Exp. Bot. 67, 2875–2888. doi: 10.1093/jxb/erw130
Almagro, M., Díaz-Pereira, E., Boix-Fayos, C., Zornoza, R., Sánchez-Navarro, V., Re, P., et al. (2023). The combination of crop diversification and no tillage enhances key soil quality parameters related to soil functioning without compromising crop yields in a low-input rainfed almond orchard under semiarid Mediterranean conditions. Agric. Ecosyst. Environ. 345, 108320. doi: 10.1016/j.agee.2022.108320
Almagro, M., Garcia-Franco, N., Martínez-Mena, M. (2017). The potential of reducing tillage frequency and incorporating plant residues as a strategy for climate change mitigation in semiarid Mediterranean agroecosystems. Agric. Ecosyst. Environ. 246, 210–220. doi: 10.1016/j.agee.2017.05.016
Altieri, M. A., Nicholls, C. I., Henao, A., Lana, M. A. (2015). Agroecology and the design of climate change-resilient farming systems. Agron. Sustain. Dev. 35, 869–890. doi: 10.1007/s13593-015-0285-2
Amarante, V., Burger, R., Chelwa, G., Cockburn, J., Kassouf, A., McKay, A., et al. (2022). Underrepresentation of developing country researchers in development research. Appl. Econ. Lett. 29, 1659–1664. doi: 10.1080/13504851.2021.1965528
Araujo, M., Ferreira de Oliveira, J. M. P., Santos, C., Moutinho-Pereira, J., Correia, C., Dias, M. C. (2019). Responses of olive plants exposed to different irrigation treatments in combination with heat shock: physiological and molecular mechanisms during exposure and recovery. Planta 249, 1583–1598. doi: 10.1007/s00425-019-03109-2
Araya-Osses, D., Casanueva, A., Román-Figueroa, C., Uribe, J. M., Paneque, M. (2020). Climate change projections of temperature and precipitation in Chile based on statistical downscaling. Clim. Dyn. 54, 4309–4330. doi: 10.1007/s00382-020-05231-4
Arenas-Castro, S., Gonçalves, J. F., Moreno, M., Villar, R. (2020). Projected climate changes are expected to decrease the suitability and production of olive varieties in southern Spain. Sci. Total Environ. 709, 136161. doi: 10.1016/j.scitotenv.2019.136161
Aria, M., Cuccurullo, C. (2017). bibliometrix: An R-tool for comprehensive science mapping analysis. J. Informetr. 11, 959–975. doi: 10.1016/j.joi.2017.08.007
Atkinson, C. J., Brennan, R. M., Jones, H. G. (2013). Declining chilling and its impact on temperate perennial crops. Environ. Exp. Bot. 91, 48–62. doi: 10.1016/j.envexpbot.2013.02.004
Barba-Espin, G., Hernandez, J. A., Diaz-Vivancos, P. (2022). Antioxidant system: The hub of bud dormancy regulation in Prunus sp. Sci. Hortic. 305, 111396. doi: 10.1016/j.scienta.2022.111396
Baronti, S., Vaccari, F. P., Miglietta, F., Calzolari, C., Lugato, E., Orlandini, S., et al. (2014). Impact of biochar application on plant water relations in Vitis vinifera (L.). Eur. J. Agron. 53, 38–44. doi: 10.1016/j.eja.2013.11.003
Barreales, D., Capitão, S., Bento, A. A., Casquero, P. A., Ribeiro, A. C. (2023). Adapting almond production to climate change through deficit irrigation and foliar kaolin application in a mediterranean climate. Atmosphere 14, 1593. doi: 10.3390/atmos14101593
Bateni, C., Ventura, M., Tonon, G., Pisanelli, A. (2021). Soil carbon stock in olive groves agroforestry systems under different management and soil characteristics. Agrofor. Syst. 95, 951–961. doi: 10.1007/s10457-019-00367-7
Benlloch-Gonzalez, M., Sanchez-Lucas, R., Benlloch, M., Fernandez-Escobar, R. (2018). An approach to global warming effects on flowering and fruit set of olive trees growing under field conditions. Sci. Hortic. 240, 405–410. doi: 10.1016/j.scienta.2018.06.054
Benmoussa, H., Ben Mimoun, M., Ghrab, M., Luedeling, E. (2018). Climate change threatens central Tunisian nut orchards. Int. J. Biometeorol. 62, 2245–2255. doi: 10.1007/s00484-018-1628-x
Ben Rouina, Y., Zouari, M., Zouari, N., Ben Rouina, B., Bouaziz, M. (2020). Olive tree (Olea europaea L. cv. Zelmati) grown in hot desert climate: Physio-biochemical responses and olive oil quality. Sci. Hortic. 261, 108915. doi: 10.1016/j.scienta.2019.108915
Blanco, V., Jose Blaya-Ros, P., Torres-Sanchez, R., Domingo, R. (2020). Influence of regulated deficit irrigation and environmental conditions on reproductive response of sweet cherry trees. Plants 9, 94. doi: 10.3390/plants9010094
Blanco, V., Zoffoli, J. P., Ayala, M. (2021). Eco-physiological response, water productivity and fruit quality of sweet cherry trees under high tunnels. Sci. Hortic. 286, 110180. doi: 10.1016/j.scienta.2021.110180
Blanke, M., Kunz, A. (2009). Effect of climate change on pome fruit phenology at Klein-Altendorf - based on 50 years of meteorological and phenological records. Erwerbs-Obstbau 51, 101–114. doi: 10.1007/s10341-009-0086-3
Bogdanovich, A. Y., Andreeva, A. P., Dobrolyubov, N. Y., Krylenko, S. V., Lipka, O. N., Semonov, S. M. (2023). Possible expansion of the climatic range of the mediterranean fruit fly being a dangerous fruit pest. Russ. Meteorol. Hydrol. 48, 72–78. doi: 10.3103/S1068373923010090
Bommarco, R., Kleijn, D., Potts, S. G. (2013). Ecological intensification: harnessing ecosystem services for food security. Trends Ecol. Evol. 28, 230–238. doi: 10.1016/j.tree.2012.10.012
Bonhomme, M., Rageau, R., Lacointe, A., Gendraud, M. (2005). Influences of cold deprivation during dormancy on carbohydrate contents of vegetative and floral primordia and nearby structures of peach buds (Prunus persica L. Batch). Sci. Hortic. 105, 223–240. doi: 10.1016/j.scienta.2005.01.015
Branchereau, C., Hardner, C., Dirlewanger, E., Wenden, B., Le Dantec, L., Alletru, D., et al. (2023). Genotype-by-environment and QTL-by-environment interactions in sweet cherry (Prunus avium L.) for flowering date. Front. Plant Sci. 14. doi: 10.3389/fpls.2023.1142974
Bruinsma, J. (2003). World agriculture: towards 2015/2030: an FAO study. 1st ed. (London: Routledge). doi: 10.4324/9781315083858
Brummer, E. C., Barber, W. T., Collier, S. M., Cox, T. S., Johnson, R., Murray, S. C., et al. (2011). Plant breeding for harmony between agriculture and the environment. Front. Ecol. Environ. 9, 561–568. doi: 10.1890/100225
Buesa, I., Caccavello, G., Basile, B., Merli, M. C., Poni, S., Chirivella, C., et al. (2019). Delaying berry ripening of bobal and tempranillo grapevines by late leaf removal in a semi-arid and temperate-warm climate under different water regimes. Aust. J. Grape Wine Res. 25, 70–82. doi: 10.1111/ajgw.2019.25.issue-1
Cabezas, J. M., Ruiz-Ramos, M., Soriano, M. A., Gabaldón-Leal, C., Santos, C. (2020). and lorite, I Identifying adaptation strategies to climate change for Mediterranean olive orchards using impact response surfaces. J. Agric. Syst. 185, 102937. doi: 10.1016/j.agsy.2020.102937
Campi, P., Gaeta, L., Mastrorilli, M., Losciale, P. (2020). Innovative soil management and micro-climate modulation for saving water in peach orchards. Front. Plant Sci. 11. doi: 10.3389/fpls.2020.01052
Campoy, J. A., Darbyshire, R., Dirlewanger, E., Quero-García, J., Wenden, B. (2019). Yield potential definition of the chilling requirement reveals likely underestimation of the risk of climate change on winter chill accumulation. Int. J. Biometeorol. 63, 183–192. doi: 10.1007/s00484-018-1649-5
Campoy, J. A., Ruiz, D., Cook, N., Allderman, L., Egea, J. (2011). Clinal variation of dormancy progression in apricot. South Afr. J. Bot. 77, 618–630. doi: 10.1016/j.sajb.2010.12.006
Cantin, C. M., Wang, X.-W., Almira, M., Arus, P., Eduardo, I. (2020). Inheritance and QTL analysis of chilling and heat requirements for flowering in an interspecific almond x peach (Texas x Earlygold) F-2 population. Euphytica 216, 51. doi: 10.1007/s10681-020-02588-9
Carvalho, L. C., Silva, M., Coito, J. L., Rocheta, M. P., Amancio, S. (2017). Design of a custom RT-qPCR array for assignment of abiotic stress tolerance in traditional portuguese grapevine varieties. Front. Plant Sci. 8. doi: 10.3389/fpls.2017.01835
Castede, S., Campoy, J. A., Quero-Garcia, J., Le Dantec, L., Lafargue, M., Barreneche, T., et al. (2014). Genetic determinism of phenological traits highly affected by climate change in Prunus avium: flowering date dissected into chilling and heat requirements. New Phytol. 202, 703–715. doi: 10.1111/nph.12658
Ceccanti, C., Landi, M., Antichi, D., Guidi, L., Manfrini, L., Monti, M., et al. (2020). Bioactive properties of fruits and leafy vegetables managed with integrated, organic, and organic no-tillage practices in the mediterranean area: A two-year rotation experiment. Agronomy 10, 841. doi: 10.3390/agronomy10060841
Cerutti, A. K., Bruun, S., Beccaro, G. L., Bounous, G. (2011). A review of studies applying environmental impact assessment methods on fruit production systems. J. Environ. Manage. 92, 2277–2286. doi: 10.1016/j.jenvman.2011.04.018
Cogato, A., Meggio, F., De Antoni Migliorati, M., Marinello, F. (2019). Extreme weather events in agriculture: A systematic review. Sustainability 11, 2547. doi: 10.3390/su11092547
Cook, R. J. (2006). Toward cropping systems that enhance productivity and sustainability. Proc. Natl. Acad. Sci. U. S. A. 103, 18389–18394. doi: 10.1073/pnas.0605946103
Costi, E., Haye, T., Maistrello, L. (2017). Biological parameters of the invasive brown marmorated stink bug, Halyomorpha halys, in southern Europe. J. Pest Sci. 90, 1059–1067. doi: 10.1007/s10340-017-0899-z
Cummings, S., Hoebink, P. (2017). Representation of academics from developing countries as authors and editorial board members in scientific journals: Does this matter to the field of development studies? Eur. J. Dev. Res. 29, 369–383. doi: 10.1057/s41287-016-0002-2
Dag, A., Harlev, G., Lavee, S., Zipori, I., Kerem, Z. (2014). Optimizing olive harvest time under hot climatic conditions of Jordan Valley, Israel. Eur. J. Lipid Sci. Technol. 116, 169–176. doi: 10.1002/ejlt.201300211
Darbyshire, R., Measham, P., Goodwin, I. (2016). A crop and cultivar-specific approach to assess future winter chill risk for fruit and nut trees. Clim. Change 137, 541–556. doi: 10.1007/s10584-016-1692-3
Darbyshire, R., Webb, L., Goodwin, I., Barlow, E. W. R. (2014). Challenges in predicting climate change impacts on pome fruit phenology. Int. J. Biometeorol. 58, 1119–1133. doi: 10.1007/s00484-013-0705-4
del Barrio, R., Fernandez, E., Brendel, A. S., Whitney, C., Campoy, J. A., Luedeling, E. (2021). Climate change impacts on agriculture’s southern frontier – Perspectives for farming in North Patagonia. Int. J. Climatol. 41, 726–742. doi: 10.1002/joc.6649
del Barrio, R. A., Orioli, G. A., Brendel, A. S., Lindström, L. I., Pellegrini, C. N., Campoy, J. A. (2022). Persian walnut (Juglans regia L.) bud dormancy dynamics in northern patagonia, Argentina. Front. Plant Sci. 12. doi: 10.3389/fpls.2021.803878
Delgado, A., Dapena, E., Fernandez, E., Luedeling, E. (2021). Climatic requirements during dormancy in apple trees from northwestern Spain-Global warming may threaten the cultivation of high-chill cultivars. Eur. J. Agron. 130, 126374. doi: 10.1016/j.eja.2021.126374
del Pozo, A., Brunel-Saldias, N., Engler, A., Ortega-Farias, S., Acevedo-Opazo, C., Lobos, G. A., et al. (2019). Climate change impacts and adaptation strategies of agriculture in Mediterranean-climate regions (MCRs). Sustainability 11, 1–16. doi: 10.3390/su11102769
Diaz-Rueda, P., Franco-Navarro, J. D., Messora, R., Espartero, J., Rivero-Nunez, C. M., Aleza, P., et al. (2020a). SILVOLIVE, a germplasm collection of wild subspecies with high genetic variability as a source of rootstocks and resistance genes for olive breeding. Front. Plant Sci. 11. doi: 10.3389/fpls.2020.00629
Diaz-Rueda, P., Franco-Navarro, J. D., Messora, R., Espartero, J., Rivero-Nunez, C. M., Aleza, P., et al. (2020b). SILVOLIVE, a germplasm collection of wild subspecies with high genetic variability as a source of rootstocks and resistance genes for olive breeding. Front. Plant Sci. 11. doi: 10.3389/fpls.2020.00629
Drogoudi, P., Kazantzis, K., Kunz, A., Blanke, M. M. (2020). Effects of climate change on cherry production in Naoussa, Greece and Bonn, Germany: adaptation strategies. Euro-Mediterr. J. Environ. Integr. 5, 12. doi: 10.1007/s41207-020-0146-5
Eccel, E., Rea, R., Caffarra, A., Crisci, A. (2009). Risk of spring frost to apple production under future climate scenarios: the role of phenological acclimation. Int. J. Biometeorol. 53, 273–286. doi: 10.1007/s00484-009-0213-8
Egea, J. A., Caro, M., Garcia-Brunton, J., Gambin, J., Egea, J., Ruiz, D. (2022). Agroclimatic metrics for the main stone fruit producing areas in Spain in current and future climate change scenarios: Implications from an adaptive point of view. Front. Plant Sci. 13. doi: 10.3389/fpls.2022.842628
Elloumi, O., Fki, M., Benmoussa, H., Feki, M., Ben Mimoun, M., Ghrab, M. (2024). Agro-climatic requirements and reproductive potentials of pistachio pollinator specimens grown in warm area in North Africa. Sci. Hortic. 325, 112649. doi: 10.1016/j.scienta.2023.112649
Elloumi, O., Ghrab, M., Kessentini, H., Ben Mimoun, M. (2013). Chilling accumulation effects on performance of pistachio trees cv. Mateur in dry and warm area climate. Sci. Hortic. 159, 80–87. doi: 10.1016/j.scienta.2013.05.004
El-Otmani, M., Chouaibi, A., Azrof, C., Bouchaou, L., Choukr-Allah, R. (2020). Response of clementine mandarin to water-saving strategies under water scarcity conditions. Water 12, 2439. doi: 10.3390/w12092439
El Yaacoubi, A., Malagi, G., Oukabli, A., Citadin, I., Hafidi, M., Bonhomme, M., et al. (2016). Differentiated dynamics of bud dormancy and growth in temperate fruit trees relating to bud phenology adaptation, the case of apple and almond trees. Int. J. Biometeorol. 60, 1695–1710. doi: 10.1007/s00484-016-1160-9
El Yaacoubi, A., Malagi, G., Oukabli, A., Hafidi, M., Legave, J.-M. (2014). Global warming impact on floral phenology of fruit trees species in Mediterranean region. Sci. Hortic. 180, 243–253. doi: 10.1016/j.scienta.2014.10.041
Espinoza-Meza, S., Ortega-Farias, S., Lopez-Olivari, R., Araya-Alman, M., Carrasco-Benavides, M. (2023). Response of fruit yield, fruit quality, and water productivity to different irrigation levels for a microsprinkler-irrigated apple orchard (cv. Fuji) growing under Mediterranean conditions. Eur. J. Agron. 145, 126786. doi: 10.1016/j.eja.2023.126786
Ezzat, A., Salama, A.-M., Szabó, S., Yaseen, A. A., Molnár, B., Holb, I. J. (2021). Deficit irrigation strategies on tree physiological and chemical properties: Treatment effects, prediction based model analyses and inter-correlations. Agronomy 11, 1361. doi: 10.3390/agronomy11071361
Fadon, E., Fernandez, E., Behn, H., Luedeling, E. (2020). A conceptual framework for winter dormancy in deciduous trees. Agronomy 10, 241. doi: 10.3390/agronomy10020241
Farsi, M., Kalantar, M., Zeinalabedini, M., Vazifeshenas, M. R. (2023). First assessment of Iranian pomegranate germplasm using targeted metabolites and morphological traits to develop the core collection and modeling of the current and future spatial distribution under climate change conditions. PloS One 18, e0265977. doi: 10.1371/journal.pone.0265977
Feng, Y., Wei, J., Zhang, G., Sun, X., Wang, W., Wu, C., et al. (2018). Effects of cooling measures on ‘Nijisseiki’ pear (Pyrus pyrifolia) tree growth and fruit quality in the hot climate. Sci. Hortic. 238, 318–324. doi: 10.1016/j.scienta.2018.05.002
Fernandes de Oliveira, A., Mameli, M. G., de Pau, L., Satta, D., Nieddu, G. (2013). Deficit irrigation strategies in Vitis vinifera L. cv. Cannonau under Mediterranean Climate. Part I - Physiological responses, growth, yield and berry composition. South Afr. J. Enol. Vitic. 34, 170–183. doi: 10.21548/34-2-1092
Fernandez, E., Caspersen, L., Illert, I., Luedeling, E. (2021). Warm winters challenge the cultivation of temperate species in South America-a spatial analysis of chill accumulation. Clim. Change 169, 28. doi: 10.1007/s10584-021-03276-w
Fernandez, E., Mojahid, H., Fadon, E., Rodrigo, J., Ruiz, D. A., Egea, J., et al. (2023). Climate change impacts on winter chill in Mediterranean temperate fruit orchards. Reg. Environ. Change 23, 7. doi: 10.1007/s10113-022-02006-x
Fernandez, E., Schiffers, K., Urbach, C., Luedeling, E. (2022). Unusually warm winter seasons may compromise the performance of current phenology models – Predicting bloom dates in young apple trees with PhenoFlex. Agric. For. Meteorol. 322, 109020. doi: 10.1016/j.agrformet.2022.109020
Fernandez, E., Whitney, C., Cuneo, I. F., Luedeling, E. (2020a). Prospects of decreasing winter chill for deciduous fruit production in Chile throughout the 21st century. Clim. Change 159, 423–439. doi: 10.1007/s10584-019-02608-1
Fernandez, E., Whitney, C., Luedeling, E. (2020b). The importance of chill model selection - a multi-site analysis. Eur. J. Agron. 119, 126103. doi: 10.1016/j.eja.2020.126103
Ferrara, R. M., Bruno, M. R., Campi, P., Camposeo, S., De Carolis, G., Gaeta, L., et al. (2024). Water use of a super high-density olive orchard submitted to regulated deficit irrigation in Mediterranean environment over three contrasted years. Irrig. Sci. 42, 57–73. doi: 10.1007/s00271-023-00892-5
Fraga, H., Pinto, J. G., Viola, F., Santos, J. A. (2020). Climate change projections for olive yields in the Mediterranean Basin. Int. J. Climatol. 40, 769–781. doi: 10.1002/joc.6237
Funes, I., Aranda, X., Biel, C., Carbó, J., Camps, F., Molina, A. J., et al. (2016). Future climate change impacts on apple flowering date in a Mediterranean subbasin. Agric. Water Manage. 164, 19–27. doi: 10.1016/j.agwat.2015.06.013
Galindo, A., Collado-Gonzalez, J., Grinan, I., Corell, M., Centeno, A., Martin-Palomo, M. J., et al. (2018). Deficit irrigation and emerging fruit crops as a strategy to save water in Mediterranean semiarid agrosystems. Agric. Water Manage. 202, 311–324. doi: 10.1016/j.agwat.2017.08.015
Galluzzi, G., Seyoum, A., Halewood, M., Noriega, I. L., Welch, E. W., Lopez Noriega, I., et al. (2020). The role of genetic resources in breeding for climate change: the case of public breeding programmes in eighteen developing countries. Plants 9, 1–19. doi: 10.3390/plants9091129
Garcia-Tejero, I., Duran-Zuazo, V. H., Jimenez-Bocanegra, J. A., Romero, R. (2011). and muriel-fernandez, J Yield response of citrus trees to different irrigation strategies in guadalquivir river basin (SW Spain). L.Acta Hortic. 922, 83–90. doi: 10.17660/ActaHortic.2011.922.10
Gargouri, K., Bentaher, H., Rhouma, A. (2012). A novel method to assess drought stress of olive tree. Agron. Sustain. Dev. 32, 735–745. doi: 10.1007/s13593-011-0078-1
Gariglio, N., Weber, M., Perreta, M., Bouzo, C., Castro, D., Martinez-Fuentes, A., et al. (2012). Chemicals applied in fall and defoliation on dormancy evolution and release in low-chill peach `Flordaking’. Agrociencia 16, 49–59. doi: 10.31285/AGRO.17.538
Garrido, A., Breia, R., Serôdio, J., Cunha, A. (2018). “Impact of the light microclimate on photosynthetic activity of grape berry (Vitis vinifera): Insights for radiation absorption mitigations’ measures,” in Theory and practice of climate adaptation. Climate change management. Eds. Alves, F., Leal Filho, W., Azeiteiro, U. (Springer, Cham), 419–441. doi: 10.1007/978-3-319-72874-2_24
Ghrab, M., Ben Mimoun, M. (2014). Effective hydrogen cyanamide (Dormex®) application for bud break, flowering and nut yield of pistachio trees CV. Mateur in warm growing areas. Exp. Agric. 50, 398–406. doi: 10.1017/S0014479713000550
Gordo, O., Sanz, J. J. (2009). Long-term temporal changes of plant phenology in the Western Mediterranean. Glob. Change Biol. 15, 1930–1948. doi: 10.1111/j.1365-2486.2009.01851.x
Granatstein, D., Kirby, E., Ostenson, H., Willer, H. (2016). Global situation for organic tree fruits. Sci. Hortic. 208, 3–12. doi: 10.1016/j.scienta.2015.12.008
Guo, L., Wang, J., Li, M., Liu, L., Xu, J., Cheng, J., et al. (2019). Distribution margins as natural laboratories to infer species’ flowering responses to climate warming and implications for frost risk. Agric. For. Meteorol. 268, 299–307. doi: 10.1016/j.agrformet.2019.01.038
Gutierrez, A. P., Ponti, L., d’Oultremont, T., Ellis, C. K. (2008). Climate change effects on poikilotherm tritrophic interactions. Clim. Change 87, S167–S192. doi: 10.1007/s10584-007-9379-4
Gutierrez, A. P., Ponti, L., Gilioli, G., Baumgärtner, J. (2018). Climate warming effects on grape and grapevine moth (Lobesia botrana) in the Palearctic region. Agric. For. Entomol. 20, 255–271. doi: 10.1111/afe.12256
Gutierrez, A. P., Ponti, L., Neteler, M., Suckling, D. M., Cure, J. R. (2021). Invasive potential of tropical fruit flies in temperate regions under climate change. Commun. Biol. 4, 1141. doi: 10.1038/s42003-021-02599-9
Gutierrez-Gamboa, G., Guerrero-Mendez, M., Araya-Alman, M., Verdugo-Vasquez, N., Valdes-Gomez, H., Acevedo-Opazo, C. (2022). Sunscreen based on dicarboxylic acid salts applications to blueberries (Vaccinium corymbosum L.) plants: Effects on water stress tolerance and productivity. Horticulturae 8, 95. doi: 10.3390/horticulturae8020095
Gutierrez-Gordillo, S., Lipan, L., Duran Zuazo, V. H., Sendra, E., Hernandez, F., Hernandez-Zazueta, M. S., et al. (2020). Deficit irrigation as a suitable strategy to enhance the nutritional composition of HydroSOS almonds. Water 12, 3336. doi: 10.3390/w12123336
Hedhly, A., Hormaza, J. I., Herrero, M. (2007). Warm temperatures at bloom reduce fruit set in sweet cherry. J. Appl. Bot. Food Qual. 81, 158–164.
Hepaksoy, S., Duman, I., Tatar, O., Kurucu, Y. (2018). Impact of climatic changes on plant production in Kucuk Menderes Basin of Ege region/Turkey. J. Environ. Prot. Ecol. 19, 1667–1677.
Hoisington, D., Khairallah, M., Reeves, T., Ribaut, J.-M., Skovmand, B., Taba, S., et al. (1999). Plant genetic resources: What can they contribute toward increased crop productivity? Proc. Natl. Acad. Sci. 96, 5937–5943. doi: 10.1073/pnas.96.11.5937
Horikoshi, H. M., Sekozawa, Y., Kobayashi, M., Saito, K., Kusano, M., Sugaya, S. (2018). Metabolomics analysis of ‘Housui’ Japanese pear flower buds during endodormancy reveals metabolic suppression by thermal fluctuation. Plant Physiol. Biochem. 126, 134–141. doi: 10.1016/j.plaphy.2018.02.028
Houston, L., Capalbo, S., Seavert, C., Dalton, M., Bryla, D., Sagili, R. (2018). Specialty fruit production in the Pacific Northwest: adaptation strategies for a changing climate. Clim. Change 146, 159–171. doi: 10.1007/s10584-017-1951-y
Ibba, K., Kassout, J., Boselli, V., Er-Raki, S., Oulbi, S., Mansouri, L. E., et al. (2023). Assessing the impact of deficit irrigation strategies on agronomic and productive parameters of Menara olive cultivar: implications for operational water management. Front. Environ. Sci. 11. doi: 10.3389/fenvs.2023.1100552
Iwata, H., Minamikawa, M. F., Kajiya-Kanegae, H., Ishimori, M., Hayashi, T. (2016). Genomics-assisted breeding in fruit trees. Breed. Sci. 66, 100–115. doi: 10.1270/jsbbs.66.100
Jose, S. (2009). Agroforestry for ecosystem services and environmental benefits: an overview. Agrofor. Syst. 76, 1–10. doi: 10.1007/s10457-009-9229-7
Kendall, A., Miles, C. A., Alexander, T. R., Scheenstra, E., LaHue, G. T. (2022). Reduced irrigation during orchard establishment conserves water and maintains yield for three cider apple cultivars. HortScience 57, 118–125. doi: 10.21273/HORTSCI16252-21
Kleijn, D., Bommarco, R., Fijen, T. P. M., Garibaldi, L. A., Potts, S. G., van der Putten, W. H. (2019a). Ecological intensification: bridging the gap between science and practice. Trends Ecol. Evol. 34, 154–166. doi: 10.1016/j.tree.2018.11.002
Kleijn, D., Bommarco, R., Fijen, T. P. M., Garibaldi, L. A., Potts, S. G., van der Putten, W. H. (2019b). Ecological intensification: Bridging the gap between science and practice. Trends Ecol. Evol. 34, 154–166. doi: 10.1016/j.tree.2018.11.002
Kliewer, W. M., Schultz, H. B. (1973). Effect of sprinkler cooling of grapevines on fruit growth and composition. Am. J. Enol. Vitic. 24, 17–26. doi: 10.5344/ajev.1973.24.1.17
Kokkotos, E., Zotos, A., Patakas, A. (2020). Evaluation of water stress coefficient ks in different olive orchards. Agronomy 10, 1594. doi: 10.3390/agronomy10101594
Kottek, M., Grieser, J., Beck, C., Rudolf, B., Rubel, F. (2006). World map of the Köppen-Geiger climate classification updated. Meteorol. Z. 15, 259–263. doi: 10.1127/0941-2948/2006/0130
Kourgialas, N. N., Karatzas, G. P. (2016). A flood risk decision making approach for Mediterranean tree crops using GIS; climate change effects and flood-tolerant species. Environ. Sci. Policy 63, 132–142. doi: 10.1016/j.envsci.2016.05.020
Koutsos, T. M., Menexes, G. C., Dordas, C. A. (2019). An efficient framework for conducting systematic literature reviews in agricultural sciences. Sci. Total Environ. 682, 106–117. doi: 10.1016/j.scitotenv.2019.04.354
Legave, J.-M., Guedon, Y., Malagi, G., El Yaacoubi, A., Bonhomme, M. (2015). Differentiated responses of apple tree floral phenology to global warming in contrasting climatic regions. Front. Plant Sci. 6. doi: 10.3389/fpls.2015.01054
Leolini, L., Moriondo, M., Fila, G., Costafreda-Aumedes, S., Ferrise, R., Bindi, M. (2018). Late spring frost impacts on future grapevine distribution in Europe. Field Crops Res. 222, 197–208. doi: 10.1016/j.fcr.2017.11.018
Letelier, L., Gaete-Eastman, C., Peñailillo, P., Moya-León, M. A., Herrera, R. (2020). Southern species from the biodiversity hotspot of Central Chile: A source of color, aroma, and metabolites for global agriculture and food Industry in a scenario of climate change. Front. Plant Sci. 11. doi: 10.3389/fpls.2020.01002
Lippmann, R., Babben, S., Menger, A., Delker, C., Quint, M. (2019). Development of wild and cultivated plants under global warming conditions. Curr. Biol. 29, R1326–R1338. doi: 10.1016/j.cub.2019.10.016
Liu, J., Islam, M. T., Sapkota, S., Ravindran, P., Kumar, P. P., Artlip, T. S., et al. (2021). Ethylene-mediated modulation of bud phenology, cold hardiness, and hormone biosynthesis in peach (Prunus persica). Plants-Basel 10, 1266. doi: 10.3390/plants10071266
Lobos, G. A., Hancock, J. F. (2015). Breeding blueberries for a changing global environment: a review. Front. Plant Sci. 6. doi: 10.3389/fpls.2015.00782
Lobos, G. A., Retamales, J. B., Hancock, J. F., Flore, J. A., Cobo, N., Del Pozo, A. (2012). Spectral irradiance, gas exchange characteristics and leaf traits of Vaccinium corymbosum L. ‘Elliott’ grown under photo-selective nets. Environ. Exp. Bot. 75, 142–149. doi: 10.1016/j.envexpbot.2011.09.006
Luedeling, E. (2012). Climate change impacts on winter chill for temperate fruit and nut production: A review. Sci. Hortic. 144, 218–229. doi: 10.1016/j.scienta.2012.07.011
Luedeling, E. (2018). Interpolating hourly temperatures for computing agroclimatic metrics. Int. J. Biometeorol. 62, 1799–1807. doi: 10.1007/s00484-018-1582-7
Luedeling, E., Gassner, A. (2012). Partial Least Squares Regression for analyzing walnut phenology in California. Agric. For. Meteorol. 158, 43–52. doi: 10.1016/j.agrformet.2011.10.020
Luedeling, E., Guo, L., Dai, J., Leslie, C., Blanke, M. M. (2013). Differential responses of trees to temperature variation during the chilling and forcing phases. Agric. For. Meteorol. 181, 33–42. doi: 10.1016/j.agrformet.2013.06.018
Luedeling, E., Steinmann, K. P., Zhang, M., Brown, P. H., Grant, J., Girvetz, E. H. (2011). Climate change effects on walnut pests in California. Glob. Change Biol. 17, 228–238. doi: 10.1111/gcb.2010.17.issue-1
Luedeling, E., Zhang, M., Girvetz, E. H. (2009a). Climatic changes lead to declining winter chill for fruit and nut trees in California during 1950-2099. PloS One 4, e6166. doi: 10.1371/journal.pone.0006166
Luedeling, E., Zhang, M., Luedeling, V., Girvetz, E. H. (2009b). Sensitivity of winter chill models for fruit and nut trees to climatic changes expected in California’s Central Valley. Agric. Ecosyst. Environ. 138, 23–31. doi: 10.1016/j.agee.2009.04.016
Luedeling, E., Zhang, M., McGranahan, G., Leslie, C. (2009c). Validation of winter chill models using historic records of walnut phenology. Agric. For. Meteorol. 149, 1854–1864. doi: 10.1016/j.agrformet.2009.06.013
Lulane, E. B., Dzikiti, S., Volschenk, T., Lotze, E., Midgley, S. J. E. (2022). Quantifying water saving benefits of fixed white protective netting in irrigated apple orchards under Mediterranean-type climate conditions in South Africa. Sci. Hortic. 305, 111439. doi: 10.1016/j.scienta.2022.111439
Luzio, A., Bernardo, S., Correia, C., Moutinho-Pereira, J., Dinis, L.-T. (2021). Phytochemical screening and antioxidant activity on berry, skin, pulp and seed from seven red Mediterranean grapevine varieties (Vitis vinifera L.) treated with kaolin foliar sunscreen. Sci. Hortic. 281, 109962. doi: 10.1016/j.scienta.2021.109962
Mairech, H., López-Bernal, Á., Moriondo, M., Dibari, C., Regni, L., Proietti, P., et al. (2020). Is new olive farming sustainable? A spatial comparison of productive and environmental performances between traditional and new olive orchards with the model OliveCan. Agric. Syst. 181, 102816. doi: 10.1016/j.agsy.2020.102816
Mairech, H., Lopez-Bernal, A., Moriondo, M., Dibari, C., Regni, L., Proietti, P., et al. (2021). Sustainability of olive growing in the Mediterranean area under future climate scenarios: Exploring the effects of intensification and deficit irrigation. Eur. J. Agron. 129, 126319. doi: 10.1016/j.eja.2021.126319
Marino, G., Di Martino, S., Roxas, A. A., Caruso, T., Ferguson, L., Barone, E., et al. (2018). Sustainability of pistachio production (Pistacia vera L.) under supplemental irrigation in a Mediterranean climate. Sci. Hortic. 241, 260–266. doi: 10.1016/j.scienta.2018.06.032
Marquez-Garcia, F., Gonzalez-Sanchez, E. J., Castro-Garcia, S., Ordonez-Fernandez, R. (2013). Improvement of soil carbon sink by cover crops in olive orchards under semiarid conditions. Influence of the type of soil and weed. Span. J. Agric. Res. 11, 335–346. doi: 10.5424/sjar/2013112-3558
Martínez-Lüscher, J., Chen, C. C. L., Brillante, L., Kurtural, S. K. (2020). Mitigating heat wave and exposure damage to “Cabernet Sauvignon” wine grape with partial shading under two irrigation amounts. Front. Plant Sci. 11, 108978. doi: 10.3389/fpls.2020.579192
Martin-Gorriz, B., Maestre-Valero, J. F., Almagro, M., Boix-Fayos, C., Martínez-Mena, M. (2020). Carbon emissions and economic assessment of farm operations under different tillage practices in organic rainfed almond orchards in semiarid Mediterranean conditions. Sci. Hortic. 261, 108978. doi: 10.1016/j.scienta.2019.108978
Martin-Gorriz, B., Soto-García, M., Martínez-Alvarez, V. (2014). Energy and greenhouse-gas emissions in irrigated agriculture of SE (southeast) Spain. Effects of alternative water supply scenarios. Energy 77, 478–488. doi: 10.1016/j.energy.2014.09.031
Matzneller, P., Götz, K.-P., Chmielewski, F.-M. (2016). Spring frost vulnerability of sweet cherries under controlled conditions. Int. J. Biometeorol. 60, 123–130. doi: 10.1007/s00484-015-1010-1
Mazis, A., Litskas, V. D., Platis, D. P., Menexes, G. C., Anagnostopoulos, C. D., Tsaboula, A. D., et al. (2021). Could energy equilibrium and greenhouse gas emissions in agroecosystems play a key role in crop replacement? A case study in orange and kiwi orchards. Environ. Sci. pollut. Res. 28, 29421–29431. doi: 10.1007/s11356-021-12774-4
Mbow, C., Smith, P., Skole, D., Duguma, L., Bustamante, M. (2014). Achieving mitigation and adaptation to climate change through sustainable agroforestry practices in Africa. Curr. Opin. Environ. Sustain. 6, 8–14. doi: 10.1016/j.cosust.2013.09.002
Measham, P. F., Quentin, A. G., MacNair, N. (2014). Climate, winter chill, and decision-making in sweet cherry production. HortScience 49, 254–259. doi: 10.21273/HORTSCI.49.3.254
Meli, S., Porto, M., Belligno, A., Bufo, S. A., Mazzatura, A., Scopa, A. (2002). Influence of irrigation with lagooned urban wastewater on chemical and microbiological soil parameters in a citrus orchard under Mediterranean condition. Sci. Total Environ. 285, 69–77. doi: 10.1016/S0048-9697(01)00896-8
Meza, F., Darbyshire, R., Farrell, A., Lakso, A., Lawson, J., Meinke, H., et al. (2023). Assessing temperature-based adaptation limits to climate change of temperate perennial fruit crops. Glob. Change Biol. 29, 2557–2571. doi: 10.1111/gcb.16601
Michalopoulos, G., Kasapi, K. A., Koubouris, G., Psarras, G., Arampatzis, G., Hatzigiannakis, E., et al. (2020). Adaptation of mediterranean olive groves to climate change through sustainable cultivation practices. Climate 8, 54. doi: 10.3390/cli8040054
Mockford, A., Urbaneja, A., Ashbrook, K., Westbury, D. B. (2023). Developing perennial wildflower strips for use in Mediterranean orchard systems. Ecol. Evol. 13, e10285. doi: 10.1002/ece3.10285
Mohamad, R. S., Verrastro, V., Al Bitar, L., Roma, R., Moretti, M., Al Chami, Z. (2016). Effect of different agricultural practices on carbon emission and carbon stock in organic and conventional olive systems. Soil Res. 54, 173–181. doi: 10.1071/SR14343
Moher, D., Liberati, A., Tetzlaff, J., Altman, D. G. (2009). Preferred reporting items for systematic reviews and meta-analyses: The PRISMA Statement. PloS Med. 6, e1000097. doi: 10.1371/journal.pmed.1000097
Montanaro, G., Tuzio, A. C., Xylogiannis, E., Kolimenakis, A., Dichio, B. (2017). Carbon budget in a Mediterranean peach orchard under different management practices. Agric. Ecosyst. Environ. 238, 104–113. doi: 10.1016/j.agee.2016.05.031
Moretti, M., Van Passel, S., Camposeo, S., Pedrero, F., Dogot, T., Lebailly, P., et al. (2019). Modelling environmental impacts of treated municipal wastewater reuse for tree crops irrigation in the Mediterranean coastal region. Sci. Total Environ. 660, 1513–1521. doi: 10.1016/j.scitotenv.2019.01.043
Moriondo, M., Leolini, L., Brilli, L., Dibari, C., Tognetti, R., Giovannelli, A., et al. (2019). A simple model simulating development and growth of an olive grove. Eur. J. Agron. 105, 129–145. doi: 10.1016/j.eja.2019.02.002
Mujahid, Z., Tounekti, T., Khemira, H. (2020). Cold plasma treatment to release dormancy and improve growth in grape buds: a promising alternative to natural chilling and rest breaking chemicals. Sci. Rep. 10, 2667. doi: 10.1038/s41598-020-59097-x
Mupambi, G., Anthony, B. M., Layne, D. R., Musacchi, S., Serra, S., Schmidt, T., et al. (2018). The influence of protective netting on tree physiology and fruit quality of apple: A review. Sci. Hortic. 236, 60–72. doi: 10.1016/j.scienta.2018.03.014
Navas-Lopez, J. F., Leon, L., Rapoport, H. F., Moreno-Alias, I., Lorite, I. J., de la Rosa, R. (2019). Genotype, environment and their interaction effects on olive tree flowering phenology and flower quality. Euphytica 215, 184. doi: 10.1007/s10681-019-2503-5
Niu, Y. H., Wang, L., Wan, X. G., Peng, Q. Z., Huang, Q., Shi, Z. H. (2021). A systematic review of soil erosion in citrus orchards worldwide. CATENA 206, 105558. doi: 10.1016/j.catena.2021.105558
Noorazar, H., Kalcsits, L., Jones, V. P., Jones, M. S., Rajagopalan, K. (2022). Climate change and chill accumulation: implications for tree fruit production in cold-winter regions. Clim. Change 171, 34. doi: 10.1007/s10584-022-03339-6
Nyamukondiwa, C., Terblanche, J. S. (2010). Within-generation variation of critical thermal limits in adult Mediterranean and Natal fruit flies Ceratitis capitata and Ceratitis rosa: thermal history affects short-term responses to temperature. Physiol. Entomol. 35, 255–264. doi: 10.1111/pen.2010.35.issue-3
Ohata, K., Togano, Y., Matsumoto, T., Uchida, Y., Kurahashi, T., Itamura, H. (2017). Selection of prune (Prunus domestica L.) cultivars suitable for the East Asian Temperate Monsoon Climate: Ripening characteristics and fruit qualities of certain prunes in a Warm Southwest Region of Japan. Hortic. J. 86, 437–446. doi: 10.2503/hortj.OKD-044
Orlandi, F., Garcia-Mozo, H., Dhiab, A. B., Galán, C., Msallem, M., Fornaciari, M. (2014). Olive tree phenology and climate variations in the Mediterranean area over the last two decades. Theor. Appl. Climatol. 115, 207–218. doi: 10.1007/s00704-013-0892-2
Paltineanu, C., Chitu, E. (2020). Climate change impact on phenological stages of sweet and sour cherry trees in a continental climate environment. Sci. Hortic. 261, 109011. doi: 10.1016/j.scienta.2019.109011
Panozzo, A., Huang, H.-Y., Bernazeau, B., Meunier, F., Turc, O., Duponnois, R., et al. (2022). Impact of olive trees on the microclimatic and edaphic environment of the understorey durum wheat in an alley orchard of the mediterranean area. Agronomy 12, 527. doi: 10.3390/agronomy12020527
Panzacchi, P., Tonon, G., Ceccon, C., Scandellari, F., Ventura, M., Zibordi, M., et al. (2012). Belowground carbon allocation and net primary and ecosystem productivities in apple trees (Malus domestica) as affected by soil water availability. Plant Soil 360, 229–241. doi: 10.1007/s11104-012-1235-2
Parajuli, R., Thoma, G., Matlock, M. D. (2019). Environmental sustainability of fruit and vegetable production supply chains in the face of climate change: A review. Sci. Total Environ. 650, 2863–2879. doi: 10.1016/j.scitotenv.2018.10.019
Parkes, H., Darbyshire, R., White, N. (2020). Chilling requirements of apple cultivars grown in mild Australian winter conditions. Sci. Hortic. 260, 108858. doi: 10.1016/j.scienta.2019.108858
Pathak, T. B., Maskey, M. L., Dahlberg, J. A., Kearns, F., Bali, K. M., Zaccaria, D. (2018). Climate change trends and impacts on California Agriculture: A detailed review. Agronomy 8, 1–27. doi: 10.3390/agronomy8030025
Pedrero, F., Grattan, S. R., Ben-Gal, A., Vivaldi, G. A. (2020). Opportunities for expanding the use of wastewaters for irrigation of olives. Agric. Water Manage. 241, 106333. doi: 10.1016/j.agwat.2020.106333
Peel, M. C., Finlayson, B. L., McMahon, T. A. (2007). Updated world map of the Köppen-Geiger climate classification. Hydrol. Earth Syst. Sci. 11, 1633–1644. doi: 10.5194/hess-11-1633-2007
Perez, V., Larranaga, N., Abdallah, D., Wunsch, A., Hormaza, J. I. (2020). Genetic diversity of local peach (Prunus persica) accessions from la palma island (Canary islands, Spain). Agronomy 10, 457. doi: 10.3390/agronomy10040457
Permanhani, M., Costa, J. M., Conceição, M. A. F., de Souza, R. T., Vasconcellos, M. A. S., Chaves, M. M. (2016). Deficit irrigation in table grape: eco-physiological basis and potential use to save water and improve quality. Theor. Exp. Plant Physiol. 28, 85–108. doi: 10.1007/s40626-016-0063-9
Phani Kumar, G., Pal Murugan, M., Murkute, A. A., Singh, S. B., Kumar, G. P., Murugan, M. P., et al. (2010). A carbon sequestration strategy involving temperate fruit crops in the trans-Himalayan region. J. Hortic. Sci. Biotechnol. 85, 405–409. doi: 10.1080/14620316.2010.11512688
Pierantozzi, P., Torres, M., Tivani, M., Contreras, C., Gentili, L., Parera, C., et al. (2020). Spring deficit irrigation in olive (cv. Genovesa) growing under arid continental climate: Effects on vegetative growth and productive parameters. Agric. Water Manage. 238, 106212. doi: 10.1016/j.agwat.2020.106212
Ponti, L., Antoniocossu, Q., Gutierrez, A. P. (2009). Climate warming effects on the Olea europaea-Bactrocera oleae system in Mediterranean islands: Sardinia as an example. Glob. Change Biol. 15, 2874–2884. doi: 10.1111/j.1365-2486.2009.01938.x
Prudencio, Á.S., Dicenta, F., Martínez-Gómez, P. (2018). Monitoring dormancy transition in almond [Prunus Dulcis (Miller)Webb] during cold and warm mediterranean seasons through the analysis of a DAM (Dormancy-Associated MADS-Box) gene. Horticulturae 4, 41. doi: 10.3390/horticulturae4040041
Prudencio, Á.S., Hoeberichts, F. A., Dicenta, F., Martínez-Gómez, P., Sánchez-Pérez, R. (2021). Identification of early and late flowering time candidate genes in endodormant and ecodormant almond flower buds. Tree Physiol. 41, 589–605. doi: 10.1093/treephys/tpaa151
Qin, Y., Wang, C., Zhao, Z., Pan, X., Li, Z. (2019). Climate change impacts on the global potential geographical distribution of the agricultural invasive pest, Bactrocera dorsalis (Hendel) (Diptera: Tephritidae). Clim. Change 155, 145–156. doi: 10.1007/s10584-019-02460-3
Rai, R., Joshi, S., Roy, S., Singh, O., Samir, M., Chandra, A. (2015). Implications of changing climate on productivity of temperate fruit crops with special reference to apple. J. Hortic. 02, 136. doi: 10.4172/2376-0354.1000135
Rana, R. S., Singh, M., Pathania, R., Upadhyay, S. K., Kalia, V. (2017). Impact of changes in climatic conditions on temperate fruit production of Himachal Pradesh. MAUSAM 68, 655–662. doi: 10.54302/mausam.v68i4
Rezgui, F., Rosati, A., Lambarraa-Lehnhardt, F., Paul, C., Reckling, M. (2024). Assessing Mediterranean agroforestry systems: Agro-economic impacts of olive wild asparagus in central Italy. Eur. J. Agron. 152, 127012. doi: 10.1016/j.eja.2023.127012
Roco, L., Engler, A., Bravo-Ureta, B., Jara-Rojas, R. (2014). Farm level adaptation decisions to face climatic change and variability: Evidence from Central Chile. Environ. Sci. Policy 44, 86–96. doi: 10.1016/j.envsci.2014.07.008
Rodriguez, A., Perez-Lopez, D., Centeno, A., Ruiz-Ramos, M. (2021). Viability of temperate fruit tree varieties in Spain under climate change according to chilling accumulation. Agric. Syst. 186, 102961. doi: 10.1016/j.agsy.2020.102961
Rodriguez-Pleguezuelo, C. R., Francia-Martinez, J. R., Franco-Tarifa, D. (2017). and duran-zuazo, V Subtropical fruit farming in Mediterranean weather hillside ecosystem: changes in vegetation. H.Ecosistemas Recur. Agropecu. 4, 499–509. doi: 10.19136/era.a4n12.1085
Rojano-Cruz, R., Martínez-Moreno, F. J., Galindo-Zaldívar, J., Lamas, F., González-Castillo, L., Delgado, G., et al. (2023). Impacts of a hydroinfiltrator rainwater harvesting system on soil moisture regime and groundwater distribution for olive groves in semi-arid Mediterranean regions. Geoderma 438, 116623. doi: 10.1016/j.geoderma.2023.116623
Rojas, G., Fernandez, E., Whitney, C., Luedeling, E., Cuneo, I. F. (2021). Adapting sweet cherry orchards to extreme weather events – Decision Analysis in support of farmers’ investments in Central Chile. Agric. Syst. 187, 103031. doi: 10.1016/j.agsy.2020.103031
Romero-Trigueros, C., Díaz-López, M., Vivaldi, G. A., Camposeo, S., Nicolás, E., Bastida, F. (2021). Plant and soil microbial community responses to different water management strategies in an almond crop. Sci. Total Environ. 778, 146148. doi: 10.1016/j.scitotenv.2021.146148
Romeu, J. F., Monforte, A. J., Sánchez, G., Granell, A., García-Brunton, J., Badenes, M. L., et al. (2014). Quantitative trait loci affecting reproductive phenology in peach. BMC Plant Biol. 14, 52. doi: 10.1186/1471-2229-14-52
Ronchail, J., Cohen, M., Alonso-Roldán, M., Garcin, H., Sultan, B., Angles, S. (2014). Adaptability of mediterranean agricultural systems to climate change: The example of the Sierra Mágina olive-growing region (Andalusia, Spain). Part II: The future. Weather Clim. Soc 6, 451–467. doi: 10.1175/WCAS-D-12-00045.1
Ronco, P., Zennaro, F., Torresan, S., Critto, A., Santini, M., Trabucco, A., et al. (2017). A risk assessment framework for irrigated agriculture under climate change. Adv. Water Resour. 110, 562–578. doi: 10.1016/j.advwatres.2017.08.003
Rosati, A., Metcalf, S. G., Buchner, R. P., Fulton, A. E., Lampinen, B. D. (2007). Effects of kaolin application on light absorption and distribution, radiation use efficiency and photosynthesis of almond and walnut canopies. Ann. Bot. 99, 255–263. doi: 10.1093/aob/mcl252
Ruiz, D., Egea, J. (2008). Analysis of the variability and correlations of floral biology factors affecting fruit set in apricot in a Mediterranean climate. Sci. Hortic. 115, 154–163. doi: 10.1016/j.scienta.2007.08.016
Ruiz, D., Garcia-Gomez, B. E., Egea, J., Molina, A., Martinez-Gomez, P., Campoy, J. A. (2019). Phenotypical characterization and molecular fingerprinting of natural early-flowering mutants in apricot (Prunus Armeniaca L.) and Japanese plum (P. salicina Lindl.). Sci. Hortic. 254, 187–192. doi: 10.1016/j.scienta.2019.05.002
Ruiz-Sánchez, M. C., Abrisqueta, I., Conejero, W., Vera, J. (2018). “Deficit irrigation management in early-maturing peach crop,” in Water scarcity and sustainable agriculture in semiarid environment (London: Elsevier), 111–129. doi: 10.1016/B978-0-12-813164-0.00006-5
Sahu, N., Saini, A., Behera, S. K., Sayama, T., Sahu, L., Nguyen, V.-T.-V., et al. (2020). Why apple orchards are shifting to the higher altitudes of the Himalayas? PloS One 15, e0235041. doi: 10.1371/journal.pone.0235041
Salama, A.-M., Ezzat, A., El-Ramady, H., Alam-Eldein, S. M., Okba, S. K., Elmenofy, H. M., et al. (2021). Temperate fruit trees under climate change: Challenges for dormancy and chilling requirements in warm winter regions. Horticulturae 7, 86. doi: 10.3390/horticulturae7040086
Sanders, C. J., Mellor, P. S., Wilson, A. J. (2010). Invasive arthropods. Rev. Sci. Tech. - Off. Int. Epizoot. 29, 273–286. doi: 10.20506/rst.issue.29.2.44
Santos, J. A. J. A., Costa, R., Fraga, H. (2017). Climate change impacts on thermal growing conditions of main fruit species in Portugal. Clim. Change 140, 273–286. doi: 10.1007/s10584-016-1835-6
Sapkota, S., Liu, J., Islam, M. T., Ravindran, P., Kumar, P. P., Sherif, S. M. (2021). Contrasting bloom dates in two apple cultivars linked to differential levels of phytohormones and heat requirements during ecodormancy. Sci. Hortic. 288, 110413. doi: 10.1016/j.scienta.2021.110413
Sarricolea, P., Herrera-Ossandon, M., Meseguer-Ruiz, Ó. (2017). Climatic regionalisation of continental Chile. J. Maps 13, 66–73. doi: 10.1080/17445647.2016.1259592
Scandellari, F., Caruso, G., Liguori, G., Meggio, F., Assunta, M. P., Zanotelli, D., et al. (2016). A survey of carbon sequestration potential of orchards and vineyards in Italy. Eur. J. Hortic. Sci. 81, 106–114. doi: 10.17660/eJHS.2016/81.2.4
Serga, S. V., Maistrenko, O. M., Rozhok, A. I., Mousseau, T. A., Kozeretska, I. A. (2015). Colonization of a temperate-zone region by the fruit fly Drosophila simulans (Diptera: Drosophilidae). Can. J. Zool. 93, 799–804. doi: 10.1139/cjz-2015-0018
Sharma, H. C. (2014). Climate change effects on insects: Implications for crop protection and food security. J. Crop Improv. 28, 229–259. doi: 10.1080/15427528.2014.881205
Shukla, I. R., Skea, J., Slade, R., Khourdajie, A. A., van Diemen, R., McCollum, D. (2022). “Climate change 2022: mitigation of climate change. Contribution of working group III to the sixth assessment report of the intergovernmental panel on climate change”. (Cambridge, UK and New York, NY, USA: Cambridge University Press). doi: 10.1017/9781009157926.026
Siakou, M., Bruggeman, A., Eliades, M., Zoumides, C., Djuma, H., Kyriacou, M. C., et al. (2021). Effects of deficit irrigation on ‘Koroneiki’ olive tree growth, physiology and olive oil quality at different harvest dates. Agric. Water Manage. 258, 107200. doi: 10.1016/j.agwat.2021.107200
Silva, S., Santo, C., Serodio, J., Silva, A. M. S., Dias, M. C. (2018). Physiological performance of drought-stressed olive plants when exposed to a combined heat-UV-B shock and after stress relief. Funct. Plant Biol. 45, 1233–1240. doi: 10.1071/FP18026
Stavi, I. (2013). Biochar use in forestry and tree-based agro-ecosystems for increasing climate change mitigation and adaptation. Int. J. Sustain. Dev. World Ecol. 20, 166–181. doi: 10.1080/13504509.2013.773466
Stephens, A. E. A., Stringer, L. D., Suckling, D. M. (2016). Advance, retreat, resettle? Climate change could produce a zero-sum game for invasive species. Austral Entomol. 55, 177–184. doi: 10.1111/aen.12162
Swarts, N., Montagu, K., Oliver, G., Southam-Rogers, L., Hardie, M., Corkrey, R., et al. (2016). Benchmarking nitrous oxide emissions in deciduous tree cropping systems. Soil Res. 54, 500–511. doi: 10.1071/SR15326
Taguas, E., Marin-Moreno, V., Diez, C. M., Mateos, L., Barranco, D., Mesas-Carrascosa, F.-J., et al. (2021). Opportunities of super high-density olive orchard to improve soil quality: Management guidelines for application of pruning residues. J. Environ. Manage. 293, 112785. doi: 10.1016/j.jenvman.2021.112785
Tanasijevic, L., Todorovic, M., Pereira, L. S., Pizzigalli, C., Lionello, P. (2014). Impacts of climate change on olive crop evapotranspiration and irrigation requirements in the Mediterranean region. Agric. Water Manage. 144, 54–68. doi: 10.1016/j.agwat.2014.05.019
Teker, T. (2023). A study of kaolin effects on grapevine physiology and its ability to protect grape clusters from sunburn damage. Sci. Hortic. 311, 111824. doi: 10.1016/j.scienta.2022.111824
Thomson, G., McCaskill, M., Goodwin, I., Kearney, G., Lolicato, S. (2014). Potential impacts of rising global temperatures on Australia’s pome fruit industry and adaptation strategies. N. Z. J. Crop Hortic. Sci. 42, 21–30. doi: 10.1080/01140671.2013.838588
Torralba, M., Fagerholm, N., Burgess, P. J., Moreno, G., Plieninger, T. (2016). Do European agroforestry systems enhance biodiversity and ecosystem services? A meta-analysis. Agric. Ecosyst. Environ. 230, 150–161. doi: 10.1016/j.agee.2016.06.002
Torres, N., Goicoechea, N., Carmen Antolín, M. (2018). Influence of irrigation strategy and mycorrhizal inoculation on fruit quality in different clones of Tempranillo grown under elevated temperatures. Agric. Water Manage. 202, 285–298. doi: 10.1016/j.agwat.2017.12.004
Tscharntke, T., Clough, Y., Bhagwat, S. A., Buchori, D., Faust, H., Hertel, D., et al. (2011). Multifunctional shade-tree management in tropical agroforestry landscapes - a review. J. Appl. Ecol. 48, 619–629. doi: 10.1111/j.1365-2664.2010.01939.x
Valverde, P., Serralheiro, R., de Carvalho, M., Maia, R., Oliveira, B., Ramos, V. (2015). Climate change impacts on irrigated agriculture in the Guadiana river basin (Portugal). Agric. Water Manage. 152, 17–30. doi: 10.1016/j.agwat.2014.12.012
Viola, F., Caracciolo, D., Pumo, D., Noto, L. V., La Loggia, G. (2014). Future climate forcings and olive yield in a mediterranean orchard. Water 6, 1562–1580. doi: 10.3390/w6061562
Viola, F., Valerio Noto, L., Cannarozzo, M., Loggia, G. L., Porporato, A. (2012). Olive yield as a function of soil moisture dynamics. Ecohydrology 5, 99–107. doi: 10.1002/eco.208
Walkiewicz, A., Kalinichenko, K., Kubaczynski, A., Brzezinska, M., Bieganowski, A. (2020). Usage of biochar for mitigation of CO2 emission and enhancement of CH4 consumption in forest and orchard Haplic Luvisol (Siltic) soils. Appl. Soil Ecol. 156, 103711. doi: 10.1016/j.apsoil.2020.103711
Weldon, C. W., Nyamukondiwa, C., Karsten, M., Chown, S. L., Terblanche, J. S. (2018). Geographic variation and plasticity in climate stress resistance among southern African populations of Ceratitis capitata (Wiedemann) (Diptera: Tephritidae). Sci. Rep. 8, 9849. doi: 10.1038/s41598-018-28259-3
Whitaker, V. M., Knapp, S. J., Hardigan, M. A., Edger, P. P., Slovin, J. P., Bassil, N. V., et al. (2020). A roadmap for research in octoploid strawberry. Hortic. Res. 7, 33. doi: 10.1038/s41438-020-0252-1
Wolf, K., Herrera, I., Tomich, T. P., Scow, K. (2017). Long-term agricultural experiments inform the development of climate-smart agricultural practices. Calif. Agric. 71, 120–124. doi: 10.3733/ca.2017a0022
Yang, J., Huo, Z., Wang, P., Wu, D., Ma, Y., Yao, S., et al. (2021). Process-based indicators for timely identification of apricot frost disaster on the warm temperate zone, China. Theor. Appl. Climatol. 146, 1143–1155. doi: 10.1007/s00704-021-03762-2
Yi, X. K., Xu, Y. L., Gao, Z. H., Zhang, J. Y., Pan, H. F., Qi, Y. J., et al. (2020). Warm winter temperature induced changes in the dormant buds of ‘dangshansuli’ pear (Pyrus bretschneideri rehd.). Indian J. Hortic. 77, 273–278. doi: 10.5958/0974-0112.2020.00037.7
Zahoor, S., Dutt, V., Mughal, A. H., Pala, N. A., Qaisar, K. N., Khan, P. A. (2021). Apple-based agroforestry systems for biomass production and carbon sequestration: implication for food security and climate change contemplates in temperate region of Northern Himalaya, India. Agrofor. Syst. 95, 367–382. doi: 10.1007/s10457-021-00593-y
Zapata-Sierra, A. J., Manzano-Agugliaro, F. (2017). Controlled deficit irrigation for orange trees in Mediterranean countries. J. Clean. Prod. 162, 130–140. doi: 10.1016/j.jclepro.2017.05.208
Keywords: global warming, deciduous trees, warm temperate climate, adaptation strategies, sustainable agriculture, production systems, prediction models, fruit breeding
Citation: Osorio-Marín J, Fernandez E, Vieli L, Ribera A, Luedeling E and Cobo N (2024) Climate change impacts on temperate fruit and nut production: a systematic review. Front. Plant Sci. 15:1352169. doi: 10.3389/fpls.2024.1352169
Received: 07 December 2023; Accepted: 27 February 2024;
Published: 18 March 2024.
Edited by:
Oscar Vicente, Universitat Politècnica de València, SpainReviewed by:
Dimitrios J. Bilalis, Agricultural University of Athens, GreeceFrancesco Maldera, University of Bari Aldo Moro, Italy
José Manuel Moutinho-Pereira, University of Trás-os-Montes and Alto Douro, Portugal
Copyright © 2024 Osorio-Marín, Fernandez, Vieli, Ribera, Luedeling and Cobo. This is an open-access article distributed under the terms of the Creative Commons Attribution License (CC BY). The use, distribution or reproduction in other forums is permitted, provided the original author(s) and the copyright owner(s) are credited and that the original publication in this journal is cited, in accordance with accepted academic practice. No use, distribution or reproduction is permitted which does not comply with these terms.
*Correspondence: Nicolas Cobo, nicolas.cobo@ufrontera.cl