(1)
Canberra, Aust Capital Terr, Australia
Scientific Name
Cynara cardunculus L.
Synonyms
Carduus cardunculus (L.) Baill., Carduus cynara E.H.L. Krause, Carduus scolymus Baill., Cnicus communis Lam., Cynara cardunculus var. altilis DC., Cynara cardunculus var. elata Cavara, Cynara cardunculus var. ferocissima Lowe, Cynara cardunculus var. inermis DC., Cynara cardunculus var. scolymus (L.) Benth., Cynara cardunculus var. sylvestris (Lam.) Fiori, Cynara corsica Viv., Cynara ferox Ten. ex Steud., Cynara horrida Aiton, Cynara spinosissima J. Presl & C. Presl, Cynara sylvestris Lam.
Family
Asteraceae
Common/English Names
Garden Artichoke, Globe Artichoke, Artichoke, French Artichoke, Green Artichoke, Bur Artichoke, Artichoke Thistle, Cardoon, Cardoon Artichoke, Ground Thistle, Prickly Cardoon, Scotch Thistle, Scottish Thistle, Spanish Thistle Artichoke, Wild Artichoke, Wild Cardoon, Wood Artichoke, Vegetable Rennet
Vernacular Names
For Globe Artichoke
Albanian: Angjinare
Arabic: Ardi Shauki, El-Kharshűf
Brazil: Alcahofra
Catalan: Carxofa, Carxofera, Escarxofer, Escarxofera
Chinese: Cai Ji, Chao Xian Ji, Chu-Chi, Chui Sin Kai (Cantonese), Ju Ji, Yang Ji
Corsican: Carciofu
Croatian: Artićok, Artičoka, Dragušica, Italijanski Osat, Osat Talijanski, Oset Pitomi, Ratićok
Czech: Artyčok, Artyčok Zeleninový
Danish: Artiskok, Artiskokblad, Artiskokker
Dutch: Artisjok, Artisjokken
Eastonian: Harilik Artišokk
Esperanto: Artiŝoko
Finnish: Artisokka, Latva-Artisokka
French: Artichaut, Artichaut Commun, Artichaut Scolyme
German: Artischocke
Greek: Agginara, Agginares, Agkinara, Agkinares, Κινάρα
Hebrew: Artishok, Kinras Tarbuti
Hungarian: Articsóka, articsóka level, Kerti Articsóka
Icelandic: Ætiþistill, Körfukál
India: Hatichu (Hindi)
Italian: Articiocco, Carciofo, Carciofolo
Japanese: Chousen Azami
Latvian: Artišoka, Artišoka Lapas
Lithuanian: Tikrasis Artišokas
Maltese: Qaqoċċ, Qaqoċċ Tax-Xewk
Nahuatl: Quahtlahuitzquilitl
Norwegian: Artisjokk
Polish: Karczoch Zwyczajny
Portuguese: Alcachofra, Alcachofra De Comer
Romanian: Anghinară
Russian: Artishok Koliuchii, Artišok Posvenoj
Spanish: Alcachofa, Alcachofera, Alcacil, Alcancil, Alcaucil, Alearrhofa, Capacaballo, Hierba De Cuajo
Slovašcina: Artičoka
Slovencina: Artičoka Zeleninová
Swedish: Kronärtskocka
Turkish: Enginar
Vietnamese: Atisô
Welsh: March-Ysgall
For Cardoon
Chinese: Ci Cai Ji
Croatian: Gardun
Czech: Artyčok Kardový
Danish: Kardon
Dutch: Kardoen
Espernato: Kardono
Finnish: Kardoni
French: Artichaut Carde, Artichaut Épineux, Artichaut Sauvage, Cardon, Cardon D’espagne Chard, Chardonnette, Chardounette
German: Cardoon, Cardy, Cynara, Gemüseartischocke, Karde, Kardone, Spanische Artichjocke, Stachelige Gemüseartischocke, Stachelige Kardone
Greek: Agria Agkinara, Agries Agginares
Hungarian: Bogáncsos Articsóka, Kárdi, Spanyol Articsóka
Italian: Caglio, Cardo, Cardoncelle, Cardone, Cardonnette, Cardoon Artichoke, Cardo Spinoso, Carduccio, Carduni
Maltese: Qaqocc Tax-Xewk
Polish: Kard, Karczoch Hiszpański
Portuguese: Cardo, Cardo Coalhador, Cardo Comestible, Cardo De Espanha, Cardo Hortense, Cardo Manso, Cardo-Da-Gente, Cardo-Do-Coalho, Cardo De Comer, Coalho, Pencas, Pencas De Cardo
Portuguese: Alcachofra Hortense Cardo
Slovašcina: Mala Artičoka
Spanish: Alcachofa, Alcaucil, Alcaucil Silvestre, Capacaballo, Cardo, Cardo De Arrecife, Cardo De Comer
Swedish: Kardon
Turkish: Yabani Enginar
Origin/Distribution
Cynara cardunculus is native to the Mediterranean and Macaronesia. Cladistic studies based on morphological character of a large set of specimens by Wiklund (1992) confirmed the inclusion of cultivated artichoke, leafy cardoon and wild cardoon in a single species: Cynara cardunculus L. The wild perennial taxon, Cynara cardunculus var. sylvestris (Lamk) Fiori, has been recognized as the ancestor of both the globe artichoke [var. sativa Moris, var. scolymus (L.) Fiori, ssp. scolymus (L.) Hegi] and the leafy or cultivated cardoon (var. altilis DC) (Rottenberg and Zohary 1996). Recent studies on isozymes and molecular markers such as RAPDs and AFLPs (Rottenberg et al. 1996; Sonnante et al. 2002, 2004; Raccuia et al. 2004a) have confirmed that both crops evolved from the wild cardoon gene pool, which can therefore be considered the progenitor of both of them. Cynara cardunculus has been divided into two subspecies: the western morph is subsp. flavescens mainly found in Macaronesia, Portugal and the northwest Mediterranean region; the eastern morph is subsp. cardunculus found mainly in central to northeast Mediterranean region (Wiklund 1992). The subspecies differ mainly by a yellowish margin on the middle involucral bract (Wiklund 1992). There are also a number of varieties of C. cardunculus including the cultivated artichoke var. scolymus (L.) Fiori, the wild artichoke var. sylvestris (Lam.) Fiori, and the leafy cardoon var. altilis DC (Pignone and Sonnante 2004).
Globe artichoke is widely cultivated in the Mediterranean area, chiefly in Spain, Italy and France, also in the southern United States, South America, China, Egypt, Morocco, and elsewhere including Vietnam, in the Dalat Highlands where it is grown mainly for producing artichoke tea and also as vegetables. In the United States, California provides more than 90 % of the US Crop.
Agroecology
Globe artichoke is a cool-season crop (Basnizki 1985; Bianco 1990; Bratsch 2009), with a wide adaptive range of 7–30 °C. It grows best in areas with day temperatures of 20–22 °C and 12–14 °C in the night, representing the optimal values to obtain compact and tender heads for an extended period. The minimal biological temperature ranges between 7 and 9 °C, while the lethal one is lesser than −10 °C (Bianco 1990). Frosts damage outer portions of the buds; severe or frequent frosts damage or kill the plants. Plants are tolerant to high temperatures (>30 °C) that, however, tend to decrease the quality of edible heads. Perennial plantings are not recommended in areas where warm to hot temperatures are common. In areas with hot, dry summers, shading and mist irrigation may help vegetative growth, but hot growing conditions tend to toughen bud scales, reduce palatability and produce poor yields. New artichoke plants require ‘vernalization’ or ‘chilling’ (Bratsch 2009). This occurs just after planting, with seedling exposure to cool temperatures (8–10 days or of 7–10 °C). Globe artichokes are obligated long-day plants with a critical photoperiod of 10.5 hours. In seed-planted individuals, the transition from vegetative to reproductive stage depends on the interaction between the following factors (Basnizki 1985): attainment of a critical plant size (usually a rosette of seven to eight leaves), low temperatures and photoperiod. The globe artichoke are deep-rooted crops; it can be grown on a wide range of soils, but it produces best on deep, fertile, friable, well-drained soils with a pH between 6 and 8. The extremes of heavy clay and light sandy soils should be avoided (Bratsch 2009). It abhors water-logged condition and raised-bed culture is recommended where drainage is suspect.
Edible Plant Parts and Uses
The edible portion of the immature flower head (capitulum) consists primarily of the fleshy lower portions of the involucral bracts (phyllaries) and the fleshy base of the receptacle (Plates 1 and 2), known as the ‘heart’; the mass of inedible immature florets in the centre of the bud are called the ‘choke’. The lower fleshy parts of the involucral bracts (phyllaries) and the fleshy receptacle are eaten raw boiled, steamed, baked, fried, stuffed, marinated, pickled, made tino artichoke dips or mixed into yoghurt (Grieve 1971; Facciola 1990; Roberts 2000; Anoymous 2013). The young blanched chards (tender leaf stalks) are also eaten and deemed by some to equal that of cardoon chards (Grieve 1971). The bracts are removed after cooking and eaten one at a time, sometimes dipped in butter, hollandaise, mayonnaise, aioli, lemon juice or other sauces. The chards and flowering stems are eaten cooked—blanched, braised or fried, and added to sauces. The bitter young leaves are also eaten cooked (Plates 3 and 4). In France, artichoke receptacles are very popular deep fried or made into artichoke ragout (‘artichauts en ragoût’); the dried receptacles are used in soups. In Italy, artichoke hearts in oil are the usual vegetable for spring in the ‘Four Seasons’ pizza; whole artichoke can be deep fried as in the popular recipe Jewish-style artichokes or used in Italian stuffed artichokes with a stuffing mixture of bread crumbs, garlic, oregano, parsley, grated cheese and prosciutto or sausage. In Greece, a savoury and wholesome stew, ‘aginares a la polita’, is prepared with artichoke hearts, potatoes and carrots, and flavoured with onion, lemon and dill. In Spain, the more tender, younger and smaller artichokes are sprinkled with olive oil and barbecued or sauteed in olive oil with garlic, with rice as a paella, or sauteed and combined with eggs in a tortilla (frittata). Ground lamb with different spices, raisins, pine nuts and herbs are used as stuffing in artichoke hearts in Turkey, Armenia and throughout North Africa and the Middle East.
The dried flowers are used as rennet substitute for curdling/coagulating milk.
Leaves are often removed and eaten one at a time, sometimes dipped in butter, mayonnaise, aioli or other sauces. Artichokes can also be made into an herbal tea; artichoke tea is produced as a commercial product in the Dalat region of Vietnam (Plate 5). In Mexico, the flower portion is put into water and consumed as a tisane called ‘alcachofa’. Artichoke is the primary flavour of the Italian liquor Cynar. It can be served over ice as an aperitif or as a cocktail mixed with orange juice, which is especially popular in Switzerland.
Recent studies found that the incorporation of artichoke leaf and stem meal, as a source of fibre, modified significantly wheat bread textural properties for the formulations of 9 and 12 % (Frutos et al. 2008). Breads with artichoke fibre presented higher fibre content and were considered acceptable by the sensory panel, indicating this ingredient to be adequate for its use as a fibre source in wheat bread.
The blanched leaf stalks of cardoon are eaten boiled, batter fried, sautéed, braised and pickled, or used in stews and soups (Facciola 1990; Hedrick 1972). In Italy, raw strips are dipped in olive oil or bagna cauda, a hot, anchovy and garlic dip. The tender, fleshy root can be cooked like carrot or parsnip and has an agreeable flavour. Flower-head receptacle is eaten like for artichoke. Flowers of Cynara cardunculus (cardoon) have been traditionally used as coagulants in Portugal and Spain for many centuries to produce traditional artisanal cheeses (Cordeiro et al. 1998; Sidrach et al. 2005). Aspartic proteinases from flowers of Cynara cardunculus have been extensively studied and long used as coagulants in the manufacture of several traditional Spanish and Portuguese cheeses (Sidrach et al. 2005). Today, the Serpa and Serra cheeses are some examples of typical and highly appreciated products of Portugal.
Botany
An erect, spiny, biennial or perennial herb growing to 1–2 m high by 1 m wide (Plate 1) with a fleshy taproot. Young growth is arachnoid tomentose and stems short and striate ribbed. Leaves (Plates 1, 3, 4, and 6) are rosulate on young plants; alternate on stems and on short, rigid petioles; ovate to lanceolate in outline; large, 30–50 cm long by 10–20 cm wide; pinnatifid or parted; silvery glaucous-green; pubescent to glabrescent above greyish-tomentose below; segments lanceolate and spiny; caudate; base sessile; and decurrent into wings on the stem (Plates 1 and 6). Flower purple-blue; all discoid in a loose, large (6–10 cm across the base) ovate capitula (involucre) (Plates 2, 3 and 4); and terminal on a long flowering stem to 1.5 m (Plates 6 and 7). Phyllaries broadly ovate, bases adpressed, spiny apex, green or tinged with purple or wholly purple, base fleshy, 1–5 cm long by 1–2 cm across the base, receptacle flat, and 2–2.5 cm diameter surrounding numerous tubular florets. Corollas blue or purple, 3–5 cm long, membranous, apical appendages rounded, style exerted. Achene, oblong, 4 angled, shiny brown, with plumose, white pappus, and 2–2.5 cm long.








Plate 1
Plant habit of globe artichoke

Plate 2
Harvest globe artichokes

Plate 3
Harvested globe artichoke and leaves for sale

Plate 4
Artichoke leaves and petioles sold as vegetables

Plate 5
Artichoke flower-head shavings used for tea

Plate 6
Artichoke plant with unopened flower head

Plate 7
Close view of fully opened mature flower head
Nutritive/Medicinal Properties
Globe artichoke is largely cultivated for its fleshy head or capitula (immature inflorescence) which accounts for 30–40 % of the fresh weight (Christakia et al. 2012). The edible portion of the head comprised the tender inner bracts and the receptacle commonly referred to as the ‘heart’, accounting for 33–55 % of the head (Ceccarelli et al. 2010). Analyses carried out in the United States (USDA 2012) reported raw, globe artichoke head to have the following proximate composition (per 100 g edible portion): water 84.94 g, energy 47 kcal (197 kJ), protein 3.27 g, total lipid 0.15 g, ash 1.13 g, carbohydrates 10.51 g, total dietary fibre 5.4 g, total sugars 0.99 g, Ca 44 mg, Fe 1.28 mg, Mg 60 mg, P 90 mg, K 370 mg, Na 94 mg, Zn 0.49 mg, Cu 0.231 mg, Mn 0.256 mg, Se 0.2 μg, vitamin C 11.7 mg, thiamine 0.072 mg, riboflavin 0.066 mg, niacin 1.046 mg, pantothenic acid 0.338 mg, vitamin B-6 0.116 mg, total folate 68 μg, choline 34.4 mg, betaine 0.2 mg, vitamin A 13 IU, vitamin E (α-tocopherol) 0.19 mg, vitamin K (phylloquinone) 14.8 μg, total saturated fatty acids 0.036 g, 12:0 (lauric acid) 0.002 g, 14:0 (myristic acid) 0.002 g, 16:0 (palmitic acid) 0.029 g, 18:0 (stearic acid) 0.003 g, total monounsaturated fatty acids 0.005 g, 18:1 undifferentiated 0.005 g, total polyunsaturated fatty acids 0.064 g, 18:2 undifferentiated 0.046 g, 18:3 undifferentiated 0.017 g, β-carotene 8 μg and lutein + zeaxanthin 464 μg.
Analyses carried out in the United States (USDA 2012) reported raw, cardoon to have the following proximate composition (per 100 g edible portion): water 94.00 g, energy 17 kcal, protein 0.7 g, total lipid 0.10 g, ash 1.13 g, carbohydrates 4.07 g, total dietary fibre 1.6 g, Ca 70 mg, Fe 0.708 mg, Mg 420 g, P 23 mg, K 400 mg, Na 170 mg, Zn 0.17 mg, vitamin C 2.0 mg, thiamine 0.020 mg, riboflavin 0.06630 mg, niacin 0.30 mg, vitamin B-6 0.116 mg, folate DFE 68 μg, total saturated fatty acids 0.011 g, total monounsaturated fatty acids 0.018 g and total polyunsaturated fatty acids 0.041 g.
Data obtained suggested that the edible parts (receptacles with inner and intermediate bracts) of flower heads of artichoke cultivars could represent a good source of health-promoting polyphenols (chlorogenic acid, coumaric acid, ferulic acid, cynarin, luteolin, apigenin) and therefore should be encouraged as a nutriceutical use, as an alternative to the more traditional phytopharmaceutical applications of leaf extracts of the species (Fratianni et al. 2007). Moreover, it was demonstrated that single polyphenols accumulate preferentially in specific parts of the heads and in specific genotypes.
Phenolic acids (caffeoylquinic acids) and flavonoids (luteolin and apigenin derivatives) were found in the immature inflorescence of globe artichoke, wild cardoon and cultivated cardoon (Pandino et al. 2010). Apigenin derivatives represented the major class in all samples investigated and highest in cardoon forms. Caffeoylquinic acids and luteolin derivatives were observed in globe artichoke only. The outer bracts of the flower head were found to have very low phenolic content (443 mg/kg dry matter (dm)) or zero hydroxycinnamic acid content; in contrast the receptacle (edible part) contained the highest amount, 1,473 mg/kg dm, and the inner bracts contained slightly lower, intermediate content (Pandino et al. 2011b). Globe artichoke is an ancient herbaceous plant native to the Mediterranean Basin. The edible part (flower head) of globe artichoke was found to be particularly rich in polyphenols; 19 phenolic compounds were determined (Lombardo et al. 2010) as follows: 1-O-caffeoylquinic acid; 3-O-caffeoylquinic acid; 5-O-caffeoylquinic acid (or chlorogenic acid); 4-O-caffeoylquinic acid; caffeic acid; 1,3-di-O-caffeoylquinic acid (or cynarin); luteolin 7-O-rutinoside; luteolin 7-O-glucoside; luteolin 7-O-glucuronide; narirutin; naringenin 7-O-glucoside; di-caffeoylquinic acid; 3,4-di-O-caffeoylquinic acid; 3,5-di-O-caffeoylquinic acid; 1,5-di-O-caffeoylquinic acid; apigenin 7-O-rutinoside; apigenin 7-O-glucoside; apigenin 7-O-glucuronide; and 4,5-di-O-caffeoylquinic acid. Apigenin 7-O-glucuronide was found to be the major flavonoid, with 6,298 mg/kg DM in ‘Romanesco clone C3’ receptacle, whereas chlorogenic acid represented the main caffeoylquinic acid, reaching 14,841 mg/kg DM in the inner bracts of ‘Violetto di Sicilia’.
In fresh marketable artichoke flower heads, only traces of free apigenin and luteolin were identified, while in badly injured heads, measurable amounts of the above flavonoids, as well as of free caffeic acid, were found to occur (Lattanzio and Van Sumere 1987). Also present are vanillic-, syringic-, p-coumaric- and ferulic acids, although caffeic acid proved to be the main phenolic component. Additionally, the latter phenolic acid increased considerably during storage of the healthy heads for 2 weeks at 20 °C or for 1 month at 4 °C, the increase at 20 °C being the most pronounced. In injured heads (internal blackening) stored for 2 weeks at 20 °C, a decrease in caffeic acid, as well as in most other phenolics, was recorded, while in badly injured heads, stored for the same period of time, less than half of the total amount of the caffeic acid present in fresh marketable heads was found. However, in injured heads stored for 1 month at 4 °C, the decrease in caffeic acid proceeded less rapidly. The main phenolic compounds in dried artichoke extracts were mono-caffeoylquinic acids (0.48–4.24 %), the most abundant, dicaffeoylquinic acid, and flavonoid contents were smaller, from 0.03 to 0.52 % (Häusler et al. 2002). The compound identified included pseudochlorogenic acid; neochlorogenic acid; chlorogenic acid; cryptochlorogenic acid; cynarin; 3,4-di-O-caffeoylquinic acid; 1,5,-di-O-caffeoylquinic acid, 3,5-di-O-caffeoylquinic acid; 4,5-di-O-caffeoylquinic acid; and cynaroside scolymoside and cynaropikrin. Three di-O-caffeoylquinic acid derivatives were isolated from the ethanol extracts of the flower heads of Cynara scolymus (Zhu et al. 2009).
Among the 22 major phenolic compounds from artichoke (Cynara scolymus) heads, juice and pomace, 11 caffeoylquinic acids and 8 flavonoids were detected (Schütz et al. 2004). Apigenin 7-O-glucuronide was found to be the major flavonoid in all samples investigated. 1,5-di-O-caffeoylquinic acid represented the major hydroxycinnamic acid, with 3,890 mg/kg in artichoke heads and 3,269 mg/kg in the pomace, whereas in the juice 1,3-di-O-caffeoylquinic acid (cynarin) was predominant, due to the isomerization during processing. Total phenolic contents of approximately 12 g/kg on a dry matter basis revealed artichoke pomace to be a promising source of phenolic compounds that might be recovered and used as natural antioxidants or functional food ingredients. Chlorogenic acid represented the major constituent in all artichoke-based dietary supplements investigated with the exception of juice derived from fresh flower heads, which exhibited a higher cynarin content (Schütz et al. 2006a) Furthermore, a distinction between products made from artichoke leaves or flower heads was possible. Caffeoylquinic acid derivatives, including chlorogenic acid, 1,3-di-O -caffeoylquinic acid (cynarin) and 1,5-di-O-caffeoylquinic acid in artichoke heads and leaves were analyzed and quantified by ultrafast liquid chromatography/tandem mass spectrometry (Shen et al. 2010). Average recoveries ranged from 92.1 to 113.2 %. Seven active polyphenolic compounds including apigenin-7-rutinoside and narirutin were purified from artichoke heads and leaves (Wang et al. 2003).
Both artichoke flower heads and leaves were found to be rich in phenolic compounds: benzoic and cinnamic derivatives, flavonoids and tannins (Lattanzio et al. 2005, 2009). The phenolic aglycons identified as soluble-bound or insoluble bound phenolics were caffeic, p-coumaric, ferulic, vanillic and syringic acids, and the flavonoids apigenin and luteolin. In addition, tannins (most abundant), hydrolyzable and condensed tannins or proanthocyanidins were also identified. The content of all identified phenolics decreased with the stages of development of plant tissues. Nevertheless, a high total phenolic content was found in artichoke by-products (about 2–3 % on dry matter basis). The cinnamoyl derivatives comprised four mono-caffeoylquinic acids (1-O-caffeoylquinic acid, 3-O-caffeoylquinic or neochlorogenic acid, 4-O-caffeoylquinic or cryptochlorogenic, and 5-O-caffeoylquinic or chlorogenic acid, the most abundant) and six di-caffeoylquinic acids (1,3-O-dicaffeoylquinic acid or cynarin; 1,4-O-dicaffeoylquinic acid; 1,5-O-dicaffeoylquinic acid; 3,4-O-dicaffeoylquinic acid; 3,5-O-dicaffeoylquinic acid; and 4,5-O-dicaffeoylquinic acid). The cynarin content was very low, while 3,5-O-dicaffeoylquinic acid and 1,5-O-dicaffeoylquinic acid were the most abundant components. Also, two flavonoids, apigenin-7-glycoside and luteolin-7-glycoside, were detected. The nomenclature of the mono-caffeoylquinic and dicaffeoylquinic acids is in accordance with the nomenclatural rules of IUPAC (1976).
Cyanidin 3,5-diglucoside; cyanidin 3-caffeoylsophoroside-5-glucoside; cyanidin 3-sophoroside; cyanidin 3-glucoside; cyanidin 3-caffeoylsophoroside; and cyanidin 3-caffeoylglucoside were identified in artichoke flowers, bracts and leaves (Aubert and Foury 1981). Besides the main anthocyanins found in artichoke heads (cyanidin 3,5-diglucoside; cyanidin 3-glucoside; cyanidin 3,5-malonyldiglucoside; cyanidin 3-(3″-malonyl)glucoside; and cyanidin 3-(6″-malonyl)glucoside), several minor compounds, namely, cyanidin 3-sophoroside; cyanidin malonylglucoside; cyanidin malonyldiglucoside; delphinidin glycoside; cyanidin malonylsophoroside; cyanidin pentoside; cyanidin 3-(6″-malonyl)glucoside; peonidin 3-O-β-glucoside; and peonidin 3-(6″-malonyl)glycoside were identified (Schütz et al. 2006c). Total anthocyanin content ranged from 8.4 to 1,705.4 mg/kg dry mass.
Of 32 aroma components of the volatile oil of cooked artichoke, the major components were the sesquiterpenes β-selinene and caryophllene (Buttery et al. 1978). Components deemed most important to the aroma included oct-1-en-3-one, hex-1-en-3-one, decanal, non-trans-2-enal, phenylacetaldehyde and eugenol. All the components identified included aliphatic alcohols (2-methylbutanol and 3-methylbutanol (0.9 %), 3-methylbut-2-en-1-ol (2.2 %), hex-cis-3-enol (3.5 %), hex-trans-2-enol (1.3 %), hexanol (2.7 %), and methyl heptanol (tentative) (2.5 %)), aliphatic aldehydes (non-trans-2-enal (0.2 %), pentanal (0.2 %), decanal (0.4 %), hexanal (0.5 %), octanal (0.2 %), nonanal (0.6 %), heptanal (0.2 %), and hex-trans-2-enal (1.6 %)), aliphatic ketones (oct-1-en-3-one (0.6 %), hex-1-en-3-one (0.8 %), pentan-2-one (0.1 %), and butan-2,3-dione (90.1 %)), aromatic and heterocylic compounds (phenylacetaldehyde (7 %), eugenol (2–5 %), pyridine (0.2 %), 2-petnylfuran (0.9 %), furfural (2 %), 2-acetythiazole (0.1 %), benzaldehyde (0.8 %), benzyl alcohol (1 %), eugenol), terpene alcohols (linalool (0.4 %), α-terpineol (0.2 %), and linalool oxide C (5-hydroxy-2,6,6-trimethyl-2-vinlytetrahydropyran) (0.5 %), and sesquiterpenes hydrocarbons (β-selinene (40 %), caryophllene (19 %) and humulene (1 %)).
The edible part of artichoke heads was reported to contain a high reducing sugar content and a high percentage of water-soluble polysaccharides (inulin), mainly concentrated in the receptacle; the inulin content may constitute 75 % of the total glucidic content (Lattanzio et al. 2009). Lattanzio et al. (2009) adapting from earlier work (Lattanzio et al. 2002) reported that the glucidic content of the edible portion of artichoke head of cv. Romanesco per fresh weight and dry weight basis were respectively as follows: glucose 0.44 %, 3.18 %; fructose 0.18 %, 1.27 %; sucrose 0.98 %, 6.98 %; and inulin 5.18 %, 37 %. In another study, glucose, fructose, sucrose and individual fructooligosaccharides (kestose, nystose, fructofuranosylnystose) were quantified in the heads of six different artichoke cultivars (Schütz et al. 2006b). The contents ranged from 12.9 to 71.7 g/kg DM for glucose, from 15.8 to 67.2 g/kg DM for fructose, and from 16.8 to 55.2 g/kg DM for sucrose in the artichoke heads. Kestose was the predominant fructooligosaccharide, followed by nystose and fructofuranosylnystose. In four cultivars fructofuranosylnystose was only detectable in traces and reached its maximum value of 3.6 g/kg DM in the cultivar Le Castel.
Two taraxastane-type hydroxy triterpenes, taraxasterol and faradiol, were isolated from the flowers (Yasukawa et al. 1996). A recombinant cyprosin, an aspartic proteinase from cardoon (Cynara cardunculus) flowers, was purified and characterized (White et al. 1999). It was found to consist of glycosylated heavy chains (34 or 32 kDa) plus associated light chains with molecular weights in the region of 14,000–18,000. It was also found to be susceptible to inhibitors of human immunodeficiency virus proteinase and particularly of rennin. Polyphenol oxidase (PPO) was purified from hearts of artichoke (Leoni et al. 1990). The best substrates for the enzyme at pH 6.0 were 5-O-caffeoylquinic acid (relative activity 100 %) and caffeic acid (relative activity 69 %). Although both ascorbic and citric acids were known to considerably improve the shelf life of artichoke heads, only ascorbic acid proved to be significantly inhibitory towards artichoke polyphenol oxidase. Polyphenol oxidase isolated from artichoke heads exhibited both monophenolase and diphenolase activities (Espin et al. 1997). Monophenolase activity of artichoke PPO was characterized using 4-hydroxyanisole as substrate with 3-methyl-2-benzothiazolinone hydrazone as a coupled nucleophile. The PPO isolated from artichoke head had a 57 kDa molecular mass (Dogan et al. 2005). The enzyme showed activity to 4-methylcatechol, pyrogallol, catechol and l-dopa but not to l-tyrosine, resorsinol and p-cresol. The optimum pH values for PPO were 5.0, 8.0 and 7.0 using 4-methylcatechol, pyrogallol and catechol as substrate, respectively. A basic heme peroxidase isoenzyme (AKPC) was purified from artichoke flowers (López-Molina et al. 2003). The enzyme was shown to be a monomeric glycoprotein, M r = 42,300. The substrate specificity of AKPC was characteristic of class III (guaiacol-type) peroxidases with chlorogenic and caffeic acids that are abundant in artichoke flowers, as particularly good substrates at pH 4.5. AKPC, when purified, were found to be a mixture of Ca2+-bound pentacoordinate high-spin (5cHS) and 6-aquo hexacoordinate high-spin (6cHS) ferric heme, and Ca2+-free 5cHS species (Hiner et al. 2003).
Leaf/Aerial Parts Phytochemicals
The amino acid composition of the leaves (g/kg) was determined as aspartic acid 5.21 g, threonine 6.14 g, serine 5.96 g, glutamic acid 9.51 g, glycine 4.17 g, alanine 5.50 g, valine 6.57 g, methionine 10.15 g, isoleucine 4.88 g, leucine 7.71 g, leucine 7.71 g, tyrosine 3.44 g, phenylalanine 6.32 g, histidine 3.19 g, lysine 7.18 g, arginine 4.99 g and total amino acids 80.84 g (Orlovskaya et al. 2007b). They also determined the mineral composition of the leaves (mg %), Na (2070), K (690), Ca (690), Mg (207) and P (207), and 16 were microelements Cu (0.345), Zn (0.414), Ag (0.0007), Mo (0.035), Li (0.207), Pb (0.069), Co (0.007), Ni (0.021), Ti (1.380), V (0.021), Cr (0.041), Fe (20.7), B (2.07), Al (13.8), Si (34.5) and Mn (2.07). The leaves and ethanol leaf extract also contained the following flavonoids (% of total phenolic compounds) (Orlovskaya et al. 2007b): luteolin-7-glucoside 35.19 %, 6.03 %; rutin 0.08 %, 6.33 %; dihydroquercetin 0.91 %, 0; vitexin 5.31 %, 0; orientin 0.46 %, 0; hyperoside 0.01 %, 7.47 %; apigenin 0, 0.89 %; hesperidin 2.33 %, 0; robinin 0, 1.27 %, respectively. The leaves and ethanol leaf extract also contained the following (% of total phenolic compounds): coumarin–4 hydroxycoumarin 0.88 %, 0; phenolic acids–gallic acid 0, 23.48 %; cichoric acid 0, 5.86 %; hydroxycinnamic acids–chlorogenic acid 0.10 %, 23.79 %; neochlorogenic acid 6.88 %, 2.38 %; caffeic acid 38.55 %, 6.30 %; ferulic acid 0, 5.54 %; phenolic glycoside–arbutin 9.31 %, 0, respectively.
From artichoke leaves the following compounds were isolated: flavonoids (apigenin, luteolin, luteolin-4′-glucoside, cynaroside, scolimoside, cosmoside, quercetin, rutin, isorhamnetin and luteolin-7-gentiobioside) (El-Negoumy et al. 1987; Hinou et al. 1989) and phenolic acids (cynarin, chlorogenic acid, caffeic acid, neochlorogenic acid, quinic acid, 4-caffeylquinic acid, 1-caffeylquinic acid (Dranik 1966), and isochlorogenic acid), along with the more uncommon scopoletin, hesperitin, hesperidoside, esculetin-6-O-β-glucoside and maritimein (Hinou et al. 1989). Glycosyl flavonoids (cynaroside and scolymoside) were found to be the major constituents, along with cynaropicrin, a sesquiterpene lactone, and the triterpene lupeol and chlorogenic acid in artichoke leaves (Noldin et al. 2003). Cynarin, the main compound described for artichoke, was detected in very low concentration. From the dried leaf powder, the following flavonoids were isolated: luteolin-7-O-α–l-rhamnosyl(1 → 6)-β-d-glucopyranoside, luteolin-7-O-β-d-glucopyranoside (cynaroside), apigenin-7-O-α-l-rhamnosyl(1 → 6)-β-d-glucopyranoside (apigenin-7-rutinoside) and apigenin-7-O-β-d-glucopyranoside (Zhu and Zhang 2004). Eight phenolic compounds were isolated from the n-butanol-soluble fraction of artichoke leaf extract: four caffeoylquinic acid derivatives, chlorogenic acid (1), cynarin (2), 3,5-di-O-caffeoylquinic acid (3) and 4,5-di-O-caffeoylquinic acid (4), and the four flavonoids, luteolin-7-rutinoside (5), cynaroside (6), apigenin-7-rutinoside (7) and apigenin-7-O-β-d-glucopyranoside (8) (Zhu et al. 2004). The main polyphenols detected in different tissues and developmental stages of 6 artichoke varietal types were chlorogenic acid, cynarin, luteolin 7-O-rutinoside and luteolin 7-O-glucoside (Negro et al. 2012). ‘Violet de Provence’ artichoke proved to retain the highest content of total phenols. Single polyphenols accumulated preferentially in specific parts of capitula. In leaves, most polyphenols were detected in the productive stage of the plant. Thirty-nine chlorogenic acids were detected in the leaves of 12 species of the Asteraceae family including Cynara scolymus (Jaiswal et al. 2011). Both chlorogenic acids based on trans– and cis-cinnamic acid substituents were identified. Assignment to the level of individual regioisomers was possible for seven caffeoylquinic acids (1–7), 11 dicaffeoylquinic acids (17–27), six feruloylquinic acids (9–14), two p-coumaroylquinic acids (15–16), two caffeoyl-feruloylquinic acids (28 and 29), four caffeoyl-p-coumaroylquinic acids (30–33), three dicaffeoyl-succinoylquinic acids (34–36), two dicaffeoyl-methoxyoxaloylquinic acids (37 and 38) and one tricaffeoylquinic acid (39). In addition, one caffeoylshikimic acid (40), one caffeoyltartaric acid (41), three dicaffeoyltartaric acids (42–44) and three caffeoyl-feruloyltartaric acids (45–47) were detected.
The carbohydrates of the leaves comprised alcohol-soluble sugars 21.34 % with traces of glucose; cold water-soluble polysaccharides 5.1 % made up of rhamnose: xylose: arabinose: mannose: glucose: galactose in the ratio of 1.0:1.4:tr: 24.6:7.3; hot water-soluble polysaccharides 0.78 % made up of rhamnose: xylose: arabinose: mannose: glucose: galactose in the ratio of 1.62:3.2:2.2:1.0:tr:2.4; pectinic substances 2.4 % made up of rhamnose: xylose: arabinose: mannose: glucose: galactose in the ratio of 4.3:1.0:2.5:0:0:1.4; hemicelluloses 2.63 % made up of rhamnose: xylose: arabinose: mannose: glucose: galactose in the ratio of tr:8.76:4.6:1.4:1.0:4.8 (Orlovskaya et al. 2007a).
In the leaves of cultivated and wild Cynara cardunculus, the flavones were the major compounds, whereas in the floral stem, caffeoylquinic acids were the most abundant (Pandino et al. 2011a). In particular, ‘Sylvestris Creta’ (var. sylvestris, wild cardoon) and ‘Violetto di Sicilia’ (var. scolymus, globe artichoke) showed the highest content of caffeoylquinic acid ∼ 95 % of the total measured polyphenols. The major compounds present in the leaf were luteolin derivatives in globe artichoke and apigenin derivatives in wild and cultivated cardoon.
From the cauline leaves of C. scolymus, cyanarin, a substance with stimulatory action on biliary secretion and cholesterinic metabolism, was isolated (Panizzi and Scarpati 1954). From artichoke leaves the following flavonoids were isolated: luteolin 7-β-glycoside and luteolin 7-β-rutinoside (Dranik et al. 1964); a flavonoid glycoside, cynarotriside, having the structure luteolin 7-[O-β-d-glucopyranosyl-(6 → 1)-O-β-l-rhamnopyranoside]-4′-O-β-d-glucopyranoside (Dranik and Chernobai 1966); luteolin, cynaroside (luteolin-7-glucoside) and scolymoside (luteolin-7-β-rutinoside) (Constantinescu et al. 1967); and apigenin, luteolin, apigenin-7-O-glucoside, cynaroside (luteolin-7-glucoside) and scolymoside (luteolin-7-β-rutinoside) (Hammouda et al. 1993). From the leaves the following phenolic acids and flavonoids were detected: chlorogenic acid, 1,3-di-O-caffeylquinic acid, cryptochlorogenic acid, neochlorogenic acid and 3,5-di-O-caffeylquinic acid; flavonoids: luteolin-4′-O-glucoside, luteolin 7-O-glucoside and luteolin 7-O-rhamnoglucoside (Bombardelli et al. 1977). The following organic acids were detected in artichoke: glyceric, malic, citric, glycolic, lactic and succinic acids (Bogaert et al. 1972). Lattanzio and Morone (1979) found that chlorogenic acid content declined from 4.4 to 1.6 % dry matter (dm). As the plant developed to the stage of differentiation of the capitulum and remained stable, a similar tendency was detected for cynaroside (0.4–0.16 % dm) and scolymoside (0.7–0.15 % dm), while the behaviour of cynarin was just the opposite, increasing gradually as the plant grew (0.01–0.05 % dm).
Hydroxymethyl acrylic acid was isolated from artichoke leaves (Bogaert et al. 1974). Two phenolic glucoside gallates, 2-methoxy-4-(2, 3-dihydroxy-propionyl)-phenyl-1-O-(6′-O-galloyl)-β-d-glucopyranoside and 4-hydroxy-3-methoxy-phenyl-1-O-(6′-O-galloyl)-β-d-glucopyranoside, were isolated from the leaves (Liu et al. 2009b). A sesquiterpenic lactone cynarolide was isolated from artichoke leaves (Drozdz 1968). Two guaiane-type sesquiterpene lactones, cynarinins A and B, together with seven known compounds, cynarascoloside C, cynaropicrin, aguerin B, grosheimin, aguerin A dehydrocynaropicrin and cynaratriol, were isolated from aerial part of Cynara scolymus (Li et al. 2005). Guaianolides, cynaropicrin and grosheimin, isolated from artichoke leaves were found to be associated with the leaf bitterness (Cravotto et al. 2005). Bioconversion of the chemicals generated 15 sesquiterpene lactone derivatives which were subjected to a taste sensory panel. Bitterness was markedly abated by either the loss of exomethylenes or the opening of the lactone ring. The bitterness of sesquiterpene lactones cynaropicrin and grosheimin derivatives was found not to be directly related to molecular polarity (Scotti et al. 2007). Cyanaropicrin, the bitter principle was found only in the green aerial parts in the stem sprouts, leaf stalks and green buds (up to 0.6 %) and in leaf lamina 0.5–4.5 % (in one case to 6.5 %) (Schneider and Thiele 2009). Young leaves contained more bitter principle than old ones. The top of the leaves showed 80 % more bitter substance than the leaf base. No bitter substance was found in the roots, completely developed blossoms and fruits. Three new guaianolides, 11-H-13-ethylsulfonylgrosheimin (grosulfeimin); 8-deoxy-11,13-dihydroxygrosheimin; and 8-deoxy-11-hydroxy-13-chlorogrosheimin and 8-epigrosheimin were isolated from the leaves (Barbetti et al. 1993). Other guaianolides, cynaropicrin, 3-deydrocynaropicrin, grosheimin, cynarolide and cynaratriol, had been isolated from artichoke (Barbetti et al. 1992; Jouany et al. 1975; Bernhard et al. 1979; Bernhard 1982). Besides cynaratriol, its derivatives 3,11,13-triacetylcynaratriol and 3,13-dibenzoyl-cynaratriol were also identified from the leaves (Bernhard 1982). A guaiane-type sesquiterpene lactone, 3β, 8α, 11β, 13-tetrahydroxy-10(14)-guaien-1α, 4β, 5α, 6βH-6α, 12-olide, and a known sesquiterpene lactone, cynarinin A, were isolated from the leaves (Liu et al. 2009a).
Apigenin 7-O-glucoside, cynarin, narirutin, gallic acid and caffeic acid were found as the main flavonoids and phenolic constituents in all parts of artichoke (flowers, leaves, roots, stems, stumps) (Thi and Park 2008). Water extraction gave higher value of total phenolic compounds and flavonoids than that by methanol or methanol:water extractions. Artichoke stem was found to be rich in non-starch polysaccharide (NSP) (∽38 g NSP/kg) and was similar to the receptacle (∽34 g NSP/kg), but bracts were heavily lignified; the stem could prove useful as sources of pectic polysaccharide-rich supplements (Femenia et al. 1998).
In commercially available artichoke leaf extract, the following compounds were identified: 8-deoxy-11-hydroxy-13-chlorogrosheimin, cryptochlorogenic acid, chlorogenic acid, neochlorogenic acid, cynarin, cynaratriol (tentatively), grosheimin, 8-deoxy-11,13-dihydroxygrosheimin, luteolin-7-O-rutinoside, luteolin-7-O-glucoside and cynaropicrin (Fritsche et al. 2002) The concentration of the compounds, namely, chlorogenic acid, cynarin and luteolin-7-O-glucoside, was in the range of 0.35–18.34, n.d.−1.02, 0.04–10.65 mg/g, respectively. Cynar opicrin, the predominant bitter artichoke leaf principle, was present at concentrations between <0.06 and 22.6 mg/g.
Ribulose-1,5-diphosphate carboxylase in artichoke leaves was separated, purified and characterized (Pacoda et al. 1985; Cardinali et al. 1986; Miceli et al. 1986). Cardinali et al. (2012) reported that the cationic peroxidase isoenzyme (CysPrx) isolated from artichoke leaves possessed some interesting properties, suggesting that CysPrx could be a considered as a potential candidate for industrial peroxidase application. In addition, from the CysPrx sequence, two full-length cDNAs, CysPrx1 and CysPrx2, differing for three amino acids, were isolated.
Seed Phytochemicals
Artichoke seeds were found to have crude protein 21.6 %, crude fibre 17.1 %, crude oil 24.05 % and ash 3.8 % (Foti et al. 1999). Artichoke seed oil was found to be an unsaturated semi-drying oil, with a high saponification value and acidic. It thus would require purification before used as edible oil. The fatty acid composition showed a high content of polyunsaturated acids (oleic acid 26.73 %, linoleic acid 58.89 %, linolenic acid 0.25 %) and to contain cholesterol (0.2 %), campesterol (12.8 %), Δ5-stigmasterol (16.2 %), β-sitosterol (45.6 %), Δ7-stigmasterol and Δ7-avenasterol ( 18.4-6.6 %). The oil had a yield of 20.5 %, free acidity (oleic acid) 11.3 %, acid value 22.4 mg/g, iodine value 108.4 g/100 g, unsaponifiable matter 0.9 %, mean molecular mass 306.2 and refractive index 1.4682 (25 °C) (Miceli and De Leo 1996).
Artichoke seed oil was found to be rich in oleic and linoleic acids (Hassanein et al. 2011). The fatty acid profile of artichoke oil comprised 14:0 (0.1 %), 16:0 (11.3 %), 16:1 (0.1 %), 18:0 (3.2 %), 18:1 n−9 (30.2 %), 18:1 n−7 (0.70 %), 18:2 (53.5 %), 20:0 (0.40 %), 20:1 (0.20 %) 24:0 (0.30 %). Among the triacylglycerols (TAGs), LLL, LLO LLP LOO and LOP were the major components in artichoke seed oil (L = linoleic, O = oleic, P = palmitic). Artichoke seed oil had a whole sterol content (as silyl derivatives) of 1.2 % of the total lipids and had high content of 5-stigmasterol (22.7 %), β-sitosterol (33.1 %) and 7-stigmasterol (25.7 %) and also possessed campesterol (9.5 %), avenasterol (6.4 %), spinasterol (2.2 %) and isofucosterol (0.4 %). The total content of free sterol (FS) and acylated sterol (AS) as 9-anthroylnitrile derivatives isolated from the oil was 390 mg/100 g oil (0.39 % of total lipid) comprising 280 mg/100 mg of free sterol and 110 mg/100 g acylated sterol. Both FS and AS showed the presence of β-sitosterol, isofucosterol,7-stigmasterol, avenasterol, spinasterol and β-stigmasterol/campesterol (inseparable pair). Artichoke oil also had high content (1,000 ppm) of free sterylglycerols (FSG) (687 ppm) and acylated sterylglycerols (ASG) (313 ppm) as 1-anthroylnitrile derivatives. It was found that β-sitosterol was the major component in both FSG and ASG fractions, followed by campe-/stigma-SG, isofuco-SG, 7-stigma-SG and spina-SG. Artichoke oil was found to have 311 ppm total tocopherols comprising 96.5 % α-tocopherol, 2.5 % γ-tocohpherol and 1.0 % δ-tocopherol.
Seeds and leaves of the artichoke had been reported to contain different constituents such as caffeoylquinic acid derivatives, mono- and di-caffeoylquinic acids, bitter sesquiterpene such as cynaratriol and cynaropicrin and flavonoids glycosides like apigenin, luteolin, cynaroside, scolymoside, quercetin and others (Georgieva et al. 2011). The content of total phenolics, chlorogenic acid and 1.5 dicaffeoyl-quinic-acid (cynarin) in germinating seeds varied considerably between different genetic lines and were higher than that reported in artichoke leaves (Ben-Hod et al. 1992). The authors concluded that compared to the traditional extraction from leaves, germinating seeds offer a richer and more dependable source for cynarin supply.
According to Maccarone et al. (1999), Cynara species grain oil especially cardoon had superior alimentary quality with a high content of oleic and linoleic acids in a balanced ratio, a good amount of α-tocopherol and low amounts of free acids, peroxides, saturated and linolenic acids. Triacylglycerols were the dominant constituents together with very little amounts of phospholipids and glycolipids. Curt et al. (2002) reported a range of 20–31.6 % seed oil content for C. cardunculus. Cynara seed oil profile was characterized in terms of major fatty acids as 10.7 % palmitic, 3.7 % stearic, 25.0 % oleic and 59.7 % linoleic.
Plant Biomass/Root Phytochemicals
At harvest the total biomass and root production, averaged for all Cynara cardunculus genotypes, were 20.4 and 9.8 t DM/ha; they were influenced by genotype (Raccuia and Melilli 2004). On average for all of the genotypes, the roots showed a total sugar content of 367 g DM/kg, with a cv. of 17.1 %; the main compound was inulin (85.0 % of total sugars). The wild cardoon ‘SR1’ showed the highest total sugar content (470 g/kg DM). On average for all of the genotypes, the total sugar and inulin yields were 3.6 and 3.0 t/ha, respectively. Studies showed that globe artichoke (Cynara cardunculus subsp. scolymus) crop for heads utilization, at the end of their harvest, gave a remaining above-ground biomass production, on average of all genotypes, of 10.3 t/ha DM with a range of 5.7 to 15.7 t/ha of DM, partitioned between leaves (53.4 %) and stalks (47.6 %) (Raccuia et al. 2004b). The root yield resulted on average of all genotypes 5.7 t/ha of DM. The total extracted sugars from roots resulted on average of all genotypes, 249 g/kg of DM, where inulin accounted for 89.4 % of total sugars. Root total sugars yield resulted on average of all genotypes 1.41 t/ha.
Artichoke By-Products/Waste Phytochemicals
Artichoke by-products such as leaves, external bracts and stems that are produced by the artichoke-processing industry represent a huge amount of discarded material (about 80–85 % of the total biomass of the plant), which could be used as a source of inulin but also of phenolics especially chlorogenic acid and 1,5-O-dicaffeoylquinic, 3,5-O-dicaffeoylquinic and 3,4-O-dicaffeoylquinic acids and should be considered as a raw material for the production of natural nontoxic food additives and neutraceuticals (Lattanzio et al. 2005, 2009; Ceccarelli et al. 2010).
In Spain, a leading artichoke producer in Europe, the residues proceeding from artichoke industry can form up to 60 % of the harvested plant material. Forty-five phenolic compounds were identified in artichoke waste (Sánchez-Rabaneda et al. 2003): gallic acid; caffeoylquinic acid 1,2, 3; dicaffeoylquinic acid 1, 2,3, 4,5, 6, 7; protocatechuic acid; esculin; chlorogenic acid; p-coumaric acid-O-glucoside isomers 1 and 2; eriodictyol-glucuronide; rutin (quercetin-3-O-rutinoside); dicaffeoylquinic acid derivative; hyperoside (quercetin-3-O-galactoside); luteolin-7-O-rutinoside; cynaroside (luteolin-7-O-glucoside); isoquercitrin (quercetin-3-O-glucoside); luteolin-7-O-glucuronide; luteolin-7-O-galactoside; naringenin-O-hexoside; avicularin (quercetin-3-O-arabinoside); isorhoifolin (apigenin-7-O-rutinoside); quercetin-O-pentoside; quercitrin (quercetin-3-O-rhamnoside); luteolin-7-O-neohesperidoside; apigenin-O-glucoside; prunin (naringenin-7-O-glucoside); naringin (naringenin-7-O-neohesperidoside); scolimoside (luteolin-7-O-rhamnoside); apigenin-7-O-glucuronide; quercetin-O-pentoside; phloridzin (phloretin-2-O-glucoside); feruloylquinic acid-O-glucoside; luteolin; quercetin; naringenin; and apigenin chrysoeriol. Caffeoylquinic and dicaffeoylquinic acids were the main compounds in the waste residue. A high molecular weight inulin was fabricated from artichoke agroindustrial wastes using environmentally benign aqueous extraction procedures (Lopez-Molina et al. 2005). The main constituent monosaccharide in artichoke inulin was fructose, and its degradation by inulinase indicated that it contained the expected beta-2,1-fructan bonds. Its FT-IR spectrum was identical to that of chicory inulin. The data indicated that artichoke inulin will be suitable for use in a wide range of food applications.
In various molecular, cellular and in-vivo test studies, artichoke (Cynara scolymus) leaf extracts showed antioxidative, hepatoprotective, antimicrobial, antiviral, anticancer, urinative, choleretic and anticholestatic effects as well as inhibitory actions on cholesterol biosynthesis and LDL oxidation (Kraft 1997; Lattanzio et al. 2009). The antidyspeptic actions ware mainly based on increased choleresis. Regarding clinical data, lipid-lowering, antiemetic, spasmolytic, choleretic and carminative effects had been described, along with good tolerance and a low incidence of side effects. The results of several clinical investigations showed the efficacy and safety of artichoke extracts (Cynara scolymus) in the treatment of hepato-biliary dysfunction and digestive complaints, such as sensation of fullness, loss of appetite, nausea and abdominal pain (Wegener and Fintelmann 1999). Earlier findings on a lipid-lowering and hepatoprotective effects were confirmed, and flavonoids and caffeoylquinic acids were reported to be mainly responsible for the observed actions. These and other pharmacological properties of artichoke are elaborated below.
Antioxidant Activity
In ABTS and DPPH tests, turmeric extract was found to be the most active, followed by artichoke and dandelion (Menghini et al. 2010). A luteolin-rich artichoke extract retarded low-density lipoprotein (LDL) oxidation in a dose-dependent manner as measured by a prolongation of the lag phase to conjugated diene formation, a decrease in the rate of propagation, and a sparing of endogenous LDL α-tocopherol during oxidation (Brown and Rice-Evans 1998). The pure aglycone, luteolin (1 μM), demonstrated an efficacy similar to that of 20 μg/ml artichoke extract in inhibiting lipid peroxidation. Luteolin-7-O-glucoside, one of the glycosylated forms in the diet, also demonstrated a dose-dependent reduction of LDL oxidation that was less effective than that of luteolin. Studies of the copper-chelating properties of luteolin-7-O-glucoside and luteolin suggested a potential role for chelation in the antioxidative effects of artichoke extract. The results demonstrated that the antioxidant activity of the artichoke extract was related in part to its constituent flavonoids which acted as hydrogen donors and metal ion chelators, and the effectiveness was further influenced by their partitioning between aqueous and lipophilic phases.
The crude flower head and leaf extracts and different phenolic fractions tested showed good antioxidant activity in the control of the oxidative damage caused by peroxyl radicals (the β-carotene/linoleate assay) (Lattanzio et al. 2005). All phenolics exhibited good antioxidant activity against peroxyl and hydroxyl radicals when assessed using the β-carotene/linoleate assay and the metmyoglobin assay. The same phenolics showed a lower activity when assessed using the deoxyribose assay. Overall, luteolin, caffeoylquinic acids and hydrolyzable tannins, being the most representative artichoke phenolics, may be considered the phenolic constituents responsible for the antioxidant properties of artichoke extracts. In addition other phenolics present in the extracts such as p-coumaric, ferulic, vanillic, syringic acids and apigenin-7-glucoside may contribute to the total antioxidant capacity of the extracts.
Artichoke leaf extract exerted a concentration-dependent inhibition of oxidative stress when human leukocytes were stimulated with agents that generated reactive oxygen species (ROS): hydrogen peroxide, phorbol-12-myristate-13-acetate (PMA) and N-formyl-methionyl-leucyl-phenylalanine (FMLP) (Perez-Garcia et al. 2000). Cynarin, caffeic acid, chlorogenic acid and luteolin, constituents of artichoke leaf extract, also exhibited a concentration-dependent inhibitory activity in the above models, contributing to the antioxidant activity of the extract in human neutrophils.
The 75 % ethanol artichoke leaf extract exhibited the highest antioxidant activity as evaluated by 2,2-diphenyl-1-picrylhydrazyl (DPPH) assay as well as yielded the largest quantity of polyphenolic compounds (Vamanu et al. 2011). The optimum concentration of 10 mg/ml gave maximum antioxidant activity of 65.15 %. The MIC values obtained using the freeze-dried extract ranged from 5.0 to 15.0 mg/ml. Total phenolic content varied from 2.12 to 30.38 mg/g of the extract. In separate studies, the ethanol extracts prepared from Cynara scolymus seeds and leaves were found to have DPPH radical scavenging activities (Georgieva et al. 2011). At any studied concentration of the seeds extract, the percent of the scavenged DPPH radicals was considerably higher than that calculated for the leaves extract. All investigated individual artichoke compounds from commercially artichoke leaf extract—chlorogenic acid, cynarin, luteolin and luteolin-7-O-glucoside—showed a remarkable antioxidative effect, chlorogenic acid being the strongest antioxidant compound (Fritsche et al. 2002).
Antioxidant phenolics were extracted from artichoke by-products: raw artichoke (RA), blanched (thermally treated) artichoke (BA) and artichoke blanching waters (ABW) by both methanol and water extractions (Llorach et al. 2002). Phenolic contents (expressed as caffeic acid derivatives) (grams per 100 g of dry extract) were 15.4 and 9.9 for RA when extracted with methanol and water, respectively; 24.3 and 10.3 for BA when extracted with methanol and water, respectively; and finally, 11.3 g of phenolics/100 ml of ABW. The artichoke extracts from industrial by-products showed a high free radical scavenging activity (versus both DPPH* and ABTS* + radicals) as well as capacity to inhibit lipid peroxidation (ferric thiocyanate method). The results suggested the possible use of artichoke extracts from industrial by-products as ingredients to functionalize foodstuffs (to decrease lipid peroxidation and to increase health-promoting properties). In another study one gram (dry matter) of artichoke was found to have a DPPH activity and a FRAP value in-vitro equivalent to those of 29.2 and 62.6 mg of vitamin C and to those of 77.9 and 159 mg of vitamin E, respectively (Jiménez-Escrig et al. 2003). Artichoke extracts showed good efficiency in the inhibition in vitro of low-density lipoprotein oxidation. Neither ferric-reducing ability nor 2,2′-azinobis(3-ethylbenzothiazolin-6-sulfonate) radical scavenging activity was modified in the rat plasma of the artichoke group with respect to the control group. Among different antioxidant enzymes measured (superoxide dismutase, glutathione peroxidase, glutathione reductase and catalase) in erythrocytes, only glutathione peroxidase activity was elevated in the artichoke group compared to the control group. 2-Aminoadipic semialdehyde, a protein oxidation biomarker, was decreased in plasma proteins and haemoglobin in the artichoke-fed group versus the control group. The results confirmed the in-vitro protective oxidative activity of artichoke in a rat model.
Studies demonstrated that artichoke polyphenolic extract protected cultured rat hepatocytes from the oxidative stress caused by glucose oxidase, comparable to the well-known antioxidant, N,N′-diphenyl-p-phenylenediamine (DPPD) (Miccadei et al. 2008). Further, the extract as well as chlorogenic acid prevented the loss of total glutathione and the accumulation of malondialdehyde. Artichoke leaf aqueous extracts exhibited a dose-dependent free radical scavenging effect of DPPH and an inhibitory effect of xanthine oxidase (Zan et al. 2013). The extract was found to contain phenolic compounds, flavonoids and saponins such as chlorogenic acid, caffeic acid, isoquercetrin and rutin.
The antioxidant capacity of cooked artichokes, measured by three different assays, enormously increased after cooking, particularly after steaming (up to 15-fold) and boiling (up to 8-fold) (Ferracane et al. 2008). Boiling increased the concentration of dicaffeoylquinic acid isomers much more than steaming or frying which showed similar patterns of dicaffeoylquinic concentrations. However, all cooking practices, particularly frying, decreased flavonoid concentration.
In double-blinded study carried out in 22 members of the Polish rowing team who were randomly assigned to a supplemented group (n = 12), receiving 1 gelatin capsule containing 400 mg of artichoke leaf extract three times a day for 5 weeks, or a placebo group (n = 10), plasma total antioxidant capacity (TAC) was significantly higher in the supplemented group than in the placebo group during restitution (Skarpanska-Stejnborn et al. 2008). Serum total cholesterol levels at the end of the study were significantly lower in the supplemented group than in the placebo group. The authors concluded that consuming artichoke leaf extract, a natural vegetable preparation of high antioxidant potential, resulted in higher plasma TAC than placebo but did not limit oxidative damage to erythrocytes in competitive rowers subjected to strenuous training.
The ethanol extract and fractions (chloroform, ethyl acetate and n-butanol) of the ethanol extract of fresh involucral bracts of cardoon, Cynara cardunculus, exhibited concentration-dependent antioxidant activity in the FRAP (ferric reducing antioxidant power) assay and scavenging of 2,2-diphenyl-1-picrylhydrazyl (DPPH) radical assays (Kukić et al. 2008).
Antidyspeptic Activity
A significant increase in bile flow was observed in anesthetized Wistar rats after acute treatment with artichoke leaf extract as well as after repeated administration (Sánchez-Rabaneda et al. 2003). The choleretic effects of artichoke extract were similar to those of the reference antidyspeptic compound dehydrocholic acid (DHCA).
In a randomized placebo-controlled double-blind cross-over study of 20 subjects, administration of a standardized, artichoke extract Hepar-SL forte was found to have a choleretic effect (Kirchhoff et al. 1994). At 120 and 150 minutes the volume of bile secreted under the active treatment was also significantly higher than under the placebo. In the placebo group, bile secretion fell below the initial level after 3 hours. An effective period of about 120–150 minutes was regarded as satisfactory to influence enzymatic digestion and the motor function of the intestine when the test substance was given postprandially. No side effects or changes in the laboratory parameters in connection with the experiment were observed. Results indicated that artichoke extract could be recommended for the treatment of dyspepsia, especially when the cause may be attributed to dyskinesia of the bile ducts or disorder in the assimilation of fat.
In postmarketing surveillance (phase IV) studies patients with non-specific gastrointestinal complaints receiving treatment with globe artichoke leaf extract (Hepar-SL forte; up to 1.92 g daily for 6 weeks (Fintelmann and Menssen 1996) or 6 months (Fintelmann and Petrowicz 1998) were monitored. In one study involving 533 patients with non-specific gastrointestinal complaints, including dyspepsia, functional biliary tract complaints, constipation and gastric irritation, seven adverse events (weakness, hunger, flatulence) were reported (1.3 % of participants), but no serious adverse events were reported (Fintelmann and Menssen 1996). In the second postmarketing surveillance study involving 203 patients with symptoms of dyspepsia who received globe artichoke leaf extract up to 1.92 g daily for up to 6 months, no adverse events were recorded (Fintelmann and Petrowicz 1998). The physicians’ overall judgement of tolerability was given as ‘good’ or ‘excellent’ in 98.5 % of cases. In another postmarketing surveillance study for artichoke leaf extract in a sub-group of patients with irritable bowel syndrome (IBS) symptoms, analysis of data revealed significant reductions in the severity of symptoms and favourable evaluations of overall effectiveness by both physicians and patients (Walker et al. 2001). Additionally, 96 % of patients rated artichoke extract as better than or at least equal to previous therapies administered for their symptoms, and the tolerability of the extract was very good.
Studies by Marakis et al. (2002) found that artichoke leaf extract showed promise in ameliorating upper gastrointestinal symptoms and improving quality of life in otherwise healthy subjects suffering from dyspepsia. Of the 516 participants, 454 completed the study. There was a significant reduction of all dyspeptic symptoms, with an average reduction of 40 % in global dyspepsia score for both dosage groups of 320 or 640 mg daily. There were no differences in the primary outcome measures between the two groups, but relief of state anxiety, a secondary outcome, was greater with the higher dosage. Health-related quality of life was significantly improved in both groups compared with baseline. The efficacy of artichoke leaf extract treatment of patients with functional dyspepsia (129 artichoke treatment, 115 placebo) was assessed in a 6-week, double-blind, randomized controlled trial (Holtmann et al. 2003). The overall symptom improvement over the 6 weeks of treatment was significantly greater with artichoke extract than with the placebo. Similarly, patients treated with artichoke extract showed significantly greater improvement in the global quality-of-life scores (NDI) compared with the placebo-treated patients.
In a subset analysis of a previous dose-ranging, open, postal study in adults (208) suffering dyspepsia, 2 months intervention of artichoke leaf extract was found to ameliorate symptoms of irritable bowel syndrome (IBS) in otherwise healthy volunteers (Bundy et al. 2004). A significant shift in self-reported usual bowel pattern away from ‘alternating constipation/diarrhoea’ towards ‘normal’ was observed. Nepean Dyspepsia Index (NDI) total symptom score significantly decreased by 41 % after artichoke treatment. Similarly, there was a significant 20 % improvement in the NDI total quality-of-life (QOL) score in the subset after treatment.
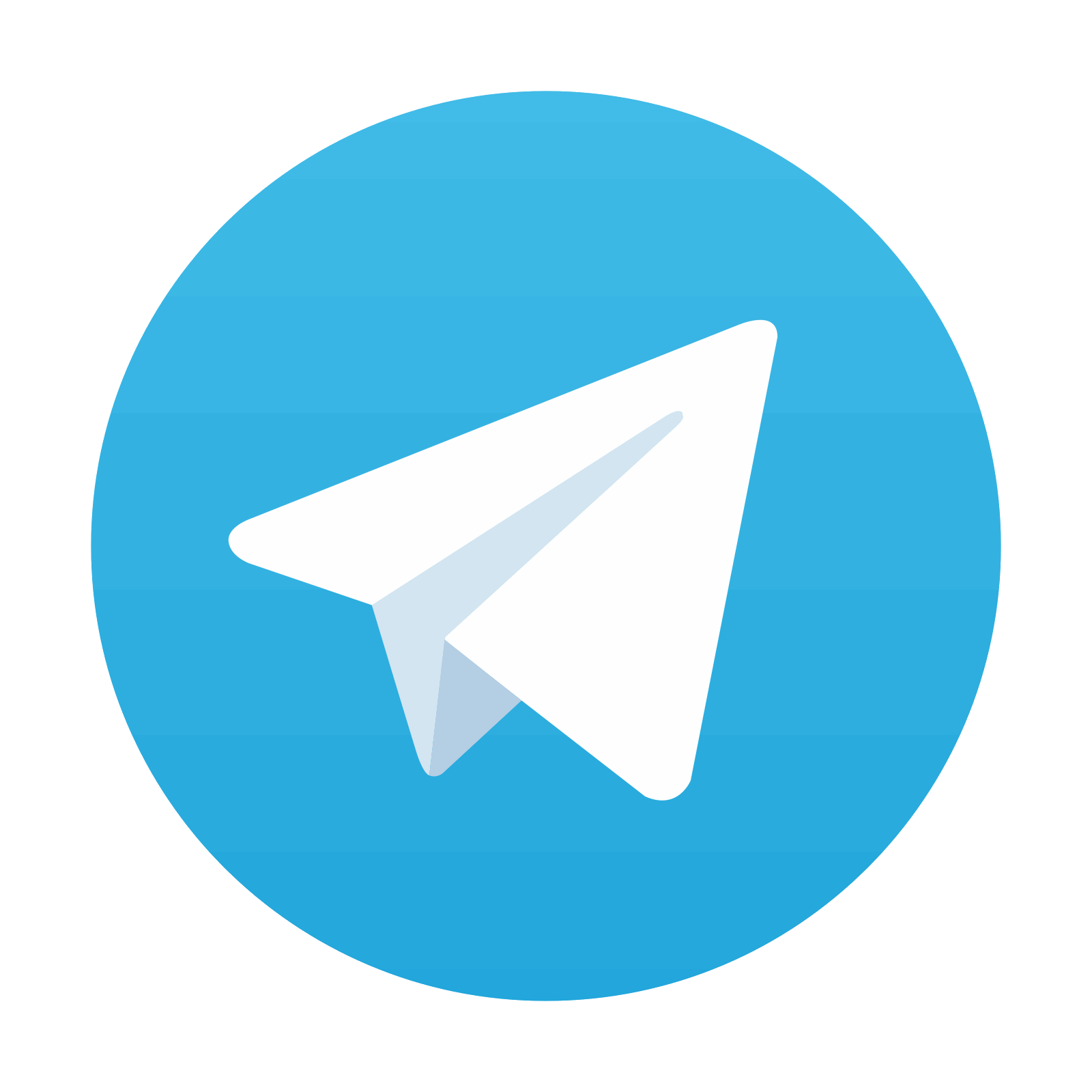
Stay updated, free articles. Join our Telegram channel

Full access? Get Clinical Tree
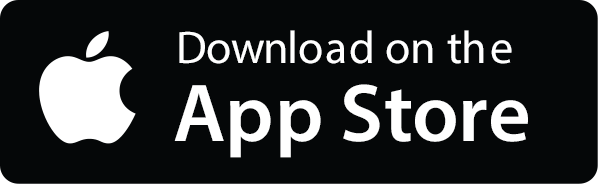
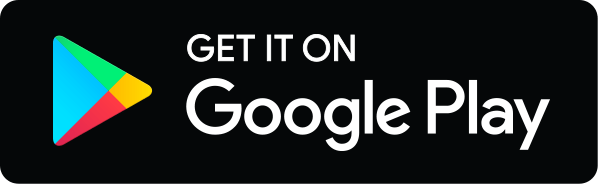